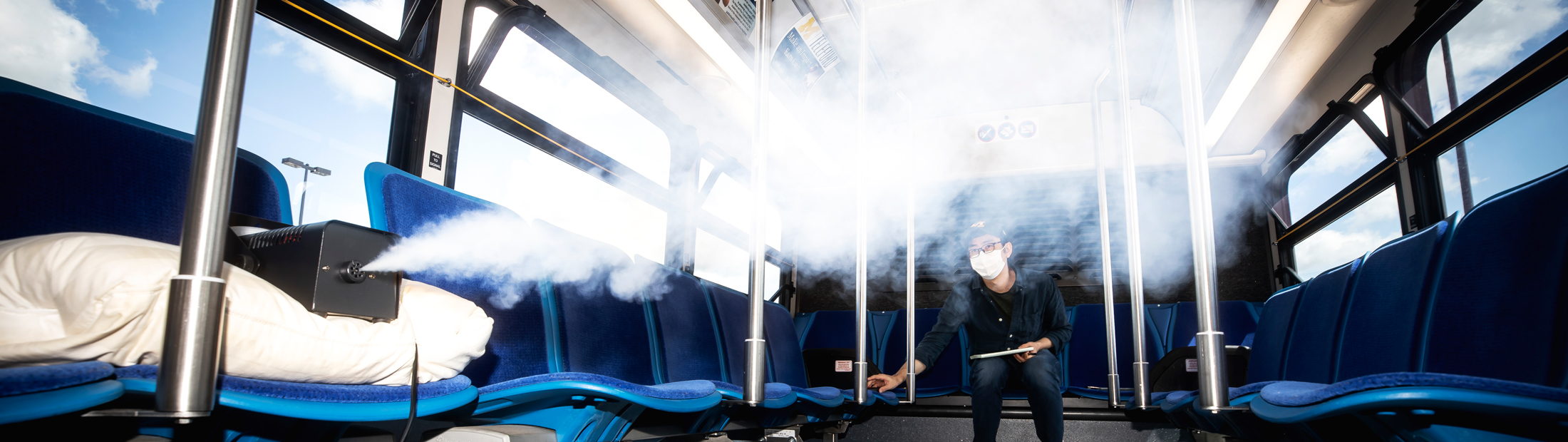
Stress test
Amid six months of tumult and chaos, engineering researchers moved quickly and collaboratively with public health officials, producing vital research in the fight against COVID-19.
Amid six months of tumult and chaos, engineering researchers moved quickly and collaboratively with public health officials, producing vital research in the fight against COVID-19.
Back in March, there was no way to anticipate the all-around dumpster fire 2020 was going to become. Theodore “Jack” Iwashyna, however, seemed to grasp part of what was coming and it was, in a word, frightening.
In a windowless North Campus conference room, late Friday afternoon on March 13, the physician and professor of internal medicine at Michigan Medicine laid out the havoc COVID-19 was already causing in hospitals around the world and, increasingly, in the United States. He also discussed how bad things were likely to get.
“We’ve had a new case roughly every day in the broader Michigan Medicine system,” he told his audience—a dozen engineers, nurses and doctors. “And you guys know, probably better than 99% of Americans, what exponential growth curves look like. Right now, we’re deceptively calm, but definitely on that exponential growth curve.”
Iwashyna had made this visit to enlist the help of Michigan Engineering in solving a variety of COVID-19-related problems facing frontline healthcare workers. And his sense of timing seemed prescient. At almost exactly the same moment the group’s first meeting was getting started, President Donald Trump was 500 miles away in Washington, D.C., declaring a national emergency due to the pandemic.
What no one in the conference room, or anywhere else for that matter, could really know at the time was everything else barreling toward the U.S. in 2020. Months of protests over social injustice and police brutality were on the way, as well as attempts by the federal government to restrict foreign students from studying at U.S. universities.
Add to this a seemingly growing percentage of the population unwilling to believe the science laid out for them, and 2020 has been something altogether…different.
COVID-19 would continue challenging the infectious disease community for the next six months and beyond. It would pose life or death questions for public health officials, governments, communities and families. Institutions like U-M would face their own version of those questions in the form of whether to bring students back to campus and how.
While classrooms, lecture halls and administrative offices across U-M were closing out of an abundance of COVID-19 caution, select engineering labs on North Campus remained open to conduct research that could directly impact the pandemic response.
In many cases, those labs welcomed new partners from completely different academic areas in order to pool expertise. That North Campus meeting spurred the first of dozens of collaborative projects between the College of Engineering and Michigan Medicine—leading to the creation of the University’s Rapid Reaction Steering Committee.
Equipment created for wholly different situations was co-opted to answer COVD-19 questions. When the equipment didn’t exist, engineers built it.
For some of them, the opportunity to work on a problem that was, quite literally, killing hundreds and thousands of people each day, brought a completely different kind of pressure to bear.
And, yet, that research, the pure science and problem-solving, as well as the collaborations it produced, may be among the few things to survive 2020 untainted.
Four days after the meeting, Margaret Wooldridge and Andre Boehman rolled equipment from their labs in G.G. Brown, out to the parking lot and into their cars for a short trip. Nearby, a mock hospital room had been set up for them to help tackle the most pressing issue raised by Iwashyna and others.
It centered around how to provide oxygen to COVID-19 patients in a way that protects them, as well as the medical professionals providing their care. There were two main options: intubation and nasal cannula.
For patients in need of higher amounts of oxygen, hospitals traditionally use intubation—a process requiring sedation and then the insertion of a breathing tube through the mouth and down into the windpipe. Ventilators then control oxygen flow.
This option forces doctors and nurses to be in close proximity to infected patients—a dangerous situation during a pandemic. In addition, intubation carries a 1% to 5% mortality risk, along with the possibility of complications, even in non-COVID-19 cases.
The other option is nasal cannula, simple air tubes placed over the nostrils. At the outset of the pandemic in the U.S., institutions had opted to go with intubation, even for patients capable of breathing with moderate oxygen assistance. The concern was that nasal cannula would spread the virus by increasing the amount of aerosolized particles emitted by ther patient. Iwashyna worried this might be wrong.
“So as of this morning, 860 Italian healthcare workers have been infected with coronavirus despite them having used a whole host of infection controls,” Iwashyna had told the group the previous week. “It’s currently deemed at many institutions that the hospital-based spread rate is unacceptably high. That means we have to move to other ways of providing oxygen.”
Iwashyna had hoped to show that heated nasal cannula could deliver oxygen without increasing virus particles projected into the air in a hospital environment. Such a finding could reduce the number of patients requiring intubation.
“If we can bring good evidence to bear on this one way or the other, whatever that answer is will have immediate public health practice implications,” he said.
To do this, Wooldridge and Boehman, both professors of mechanical engineering, reached into their bags of tricks and pulled out state-of-the-art equipment designed to measure aerosols. It was a bit of a different experience, in that their scanning mobility particle sizing systems (SMPS) and optical particle counters had previously been used to measure auto emissions.
In the simulated hospital room, two more engineering professors, Amy Cohn and Jesse Capecelatro, served as test “patients” to help measure any increases in aerosolized particles from the nasal cannula. With one SMPS affixed to the bedside rail near the patient’s head and another near their legs, the two subjects were asked to breathe normally at times and cough at others.
“Understanding how nanoparticles are transported is critical to designing the experiment, collecting the data and interpreting the results—that’s what we brought to the table,” said Wooldridge, an Arthur F. Thurnau professor of mechanical engineering. “We had never applied our expertise to this setting before, but we were happy to help.”
Wooldridge and Boehman’s equipment showed nasal cannulae do not increase the amount of virus particles exhaled, or aerosolized, by patients during treatment. Over three days of testing, measurements indicated no increase in aerosolization of particles in the range of 10 to 10,000 nanometers. A typical virus ranges in size from 20 to 400 nm.
Once results from the aerosolization testing came back, the hospital changed its policy and the research team shared their findings online.
“I got email from everybody I knew and every other division across the country, and all of them found it persuasive,” Iwashyna said. “We helped change policy across the country, almost instantaneously, to the benefit of a lot of people.
“That team saved lives.”
It didn’t take long for medical professionals on the front line to recognize COVID-19 was causing the body’s immune system to turn on itself. Cytokine storms, induced by infection, caused lungs to fill with fluid and organs to shut down, all because the body was going on the defensive.
Katsuo Kurabayashi, a U-M professor of mechanical engineering, and his research team quickly recognized an opportunity to give doctors and nurses a better way of monitoring cytokines—the proteins cells produce to signal other cells in the event of infection.
Cytokines are not detected by typical blood testing, meaning it can take hours to days for doctors to know if the levels are rising or falling, or how treatments are impacting that trend.
“The current situation here at U-M is that blood tests for cytokine levels get sent out to another laboratory and performed there and then the results get sent back to us,” said Ben Singer, a Michigan Medicine physician and expert in pulmonary and critical care, in April. “That process takes a number of days, at which point, the patient that gave that blood sample is no longer the same patient that’s in front of you.”
In March and April, Kurabayashi’s team pivoted quickly and adapted previous work focused on leukemia treatments to create a testing system capable of giving doctors accurate cytokine information, nearly in real-time. Their system, a single-molecule digital counting assay, was capable of delivering accurate cytokine levels from small amounts of blood in mere minutes.
By mid-April, the team had proven their system was effective, but the typical means of sharing their results with the medical community—publication in a peer-reviewed journal—seemed painfully slow in the midst of a public health crisis claiming so many lives each day. Their submitted paper languished with two different journals as weeks slipped by.
“It was quite the frustrating process to some degree, but not just for our paper,” Kurabayahsi said. “Because of the pandemic, a lot of things have been delayed. And many of the researchers who would normally be part of the review process are tied up with other things.
“I myself have received a lot of requests to read submitted papers. And here I am working on what I consider to be a really important project of my own. It becomes hard to find the time to spend on them.”
Eventually, the Kurabayashi team’s research was published online in medRxiv as a pre-print—a draft research paper that has not undergone peer review. It was, he said in retrospect, the best means of getting information out quickly.
“Within the COVID-19 research community, I think people were paying a lot of attention to pre-print publications,” Kurarbayashi said. “They are still cautious, but because of the urgency of collecting appropriate data, I think people were recognizing the need for having access to really critical research information.”
But speed in research can do more harm than good. The early months of the pandemic in the U.S. saw multiple high-profile, peer-reviewed papers retracted, causing confusion in the general public and providing ammunition for segments of the population that distrust the scientific community.
For something like the nasal cannula project, Iwashyna said pre-print made sense. In six weeks total time, the research went from concept to the results appearing in pre-print form online.
“It depends on the question of timeliness and who your audience is,” he said in September. “I knew the people who would be reading this were infection control specialists and people at other major universities who would review it themselves and were qualified to do it.”
Krista Wigginton picked an interesting time and place for a sabbatical. Her winter 2020 semester started in Palo Alto, where she and her family had temporarily relocated, allowing her to work with a colleague at Stanford University.
And then COVID-19 arrived, putting the U-M associate professor of civil and environmental engineering—with expertise in pathogenic viruses and detection methods—in a bit of a bind. Unable to return to Ann Arbor, she would be tasked with helping guide research on a crucial public health issue: the shortage of personal protective equipment (PPE).
“At least the weather’s nice,” she joked in March.
At that time, shortages of masks were among the top safety concerns, even though the general public was not yet wearing them. N95 masks represented the best protection widely available, but federal agencies urged the general public not to use them.
“Seriously people—STOP BUYING MASKS!” read a Tweet from the account of U.S. Surgeon General Jerome Adams just a few weeks earlier. “…if healthcare providers can’t get them to care for sick patients, it puts them and our communities at risk!”
Adams and other public health officials would eventually call for the general public to wear masks as well, but the shortage issue is one that lingers—even today.
N95 masks were intended to be a single-use product. Doctors and nurses would routinely throw them out after each visit with a patient. In early April, U-M engineers took on the challenge of extending the life of those masks.
Fortunately, Wigginton’s frequent collaborator and fellow CEE professor Nancy Love was back in Ann Arbor, along with Wigginton’s own research team and others from Michigan Medicine. That band of Ph.D., M.S. and undergraduate students was key in working out the right recipe for recycling N95 masks.
“There’s research on how coronaviruses behave in fluids, but we don’t know if they behave the same way on masks,” said Love, the Borchardt and Glysson Collegiate Professor, in March. “It’s important for experiments to be conducted to address these knowledge gaps. And few, if any, studies are doing all of the above: biological testing, mask particle reduction, and human testing.”
Working with a suite of surrogate viruses, the team looked at the effectiveness of various methods of disinfecting used masks. At the urging of Michigan Medicine, they focused on heat, ultraviolet light and hydrogen peroxide—all available at hospitals.
Researchers sprayed or placed droplets of the surrogate viruses on masks, allowing them to dry. The masks were then transported from North Campus to University Hospital where they were exposed to ultraviolet light, heat, hydrogen peroxide or a combination of the three.
The masks were returned to the engineering lab to determine how well each treatment had removed virus particles. Each mask was suspended in a solution containing proteins, then shaken to knock off particles on the material. Researchers analyzed the remaining solutions to determine how many infective viruses were left behind.
A combination of moisture and heat produced the best disinfection effect. To achieve 99.99% virus deactivation, the researchers determined that N95 masks must be heated in an oven for 20 minutes at 176 to 194 degrees Fahrenheit, with a relative humidity between 40% and 50%. Those specifications, which had not been previously determined, were easy to reproduce for medical facilities, creating an effective and accessible means of extending mask life.
“The most satisfying part of our results is how simple the solution can be,” Wigginton said. “It’s nice to know that you don’t have to necessarily invest in some super-fancy technology. Heating at 80 to 85 degrees Celsius with some added moisture is really effective.”
Wigginton also takes pride in the work her team accomplished while she was 2,000 miles away from the first half of 2020.
“At a time when most of the campus was shutting down, several members of my team worked very long, staggered hours to complete the project in a matter of weeks,” she said. “I’m proud of their dedication and organization. We not only helped develop the N95 recycling program for U-M, but ultimately produced two high quality manuscripts that we hope will help others in the future.”
Herek Clack vaguely remembers meeting Mirko Gamba years ago, maybe around the time both arrived at U-M as assistant professors in 2012. It might have been an orientation event for new faculty, or something similar, but the pair had little interaction in the years afterward.
Fast forward to 2020. Clack, a Florida-born associate professor of civil and environmental engineering, and Gamba, an associate professor of aerospace engineering originally from Italy, found themselves locked in a North Campus laboratory several nights a week for several hours at a time, for a month.
With a formula in hand for disinfecting masks, the duo took on the question of how many times masks could go through the process without losing their protective capacities. Their pairing was just one of several out-of-the box collaborations needed to address COVID-19 questions raised by the steering committee.
“It turned out our backgrounds were actually not that dissimilar,” Gamba said, looking back on the collaboration. “We had a very common foundational background, but one that took us in very different directions.
“And because it was clear what our individual strengths and weaknesses were, it was natural to form a partnership that, in my opinion, worked effectively.”
To do the testing necessary, they created a machine of their own—a windtunnel on a lab table that allowed Gamba and Clack to fire particles at decontaminated masks.
“We measure flow speed, pressure, temperature and relative humidity upstream and downstream,” Clack said in April. “Most importantly, we measure particle size distribution. Sensors take the numbers of particles counted upstream and downstream of the mask. These measures allow us to calculate the percentage of particles that penetrate the mask.”
Their work revealed decontamination methods, up to ten cycles of them, had little effect on mask integrity. It was another finding that would help stretch PPE supplies, but it also highlighted how effective new and, seemingly, odd pairings could be when brought together under the right circumstances.
“I think our core values were similar, which made us work well together immediately,” Gamba said. “And what I mean is, we were doing what we did for the same reason, and that wasn’t to have another paper published. It was to join our work in order to help however we could.”
Taken together, the decontamination formula and testing procedures represent some of Michigan Engineering’s best work during the pandemic, according to one engineer who was in the middle of many such projects.
“I think the biggest win was really the N95 reprocessing, in terms of work that involved, day-in and day-out, protecting people,” said Amy Cohn, the Arthur F. Thrunau Professor of Industrial & Operations Engineering. “We needed those masks, and getting a hold of them was a national problem.
“That was an example of so many people from so many different perspectives coming together.”
Mark Schlissel’s campus-wide email announcing a halt to in-person classes for the rest of the semester came out on March 11, just after 4 p.m. And from the moment that message went out, the clock started ticking on questions of if, when and how students would return to campus.
Universities across the globe wrestled with the question, often arriving at drastically different answers, ranging from all-remote learning to in-person classes. On June 22, Schlissel announced U-M’s plan for the fall semester, “a mixture of in-person and remote classes” likely to “look and feel different than anything we have seen before.”
Planning and preparation meant drilling down on many of the things we take for granted in campus life, including services like transportation. And U-M’s bus system is the main means of getting around Ann Arbor for many students, faculty and staff.
From 2017 through 2019, U-M’s buses provided an average of 7.6 million rides each fiscal year. For students who live on North Campus, or others who need to get there, they are crucial.
At the start of summer, a group of researchers quickly assembled to evaluate the safety of busing during the pandemic and came up with a host of suggested changes for the fall.
They studied the way particles exhaled by passengers circulate inside the vehicles under varied conditions. Another group with varied backgrounds—naval architecture and marine engineering, to industrial operations engineering—came together for the job, but had little baseline information to work with.
“We’ve really only been learning about this virus for the last few months, and in academic terms, that’s a split second,” said Jesse Capecelatro, an assistant professor of mechanical engineering specializing in messy turbulent flows.
Andre Boehman then re-entered the picture with the equipment he normally uses for auto emissions. In place of aerosols exhaled by passengers, Boehman used a theatrical smoke machine to track dispersion and dilution onboard U-M buses.
“The combination of test instruments and the simple ‘fog machine’ allowed us the flexibility to generate and measure an aerosol with a broad size range, and to release and detect that aerosol in a variety of locations within the buses,” Boehman said. “ In this way, we could quickly see how aerosols move within the bus and are diluted by mixing with air within the bus and by the addition of fresh air into the bus.”
Taking those modeling estimates and experimental data, the research team simulated how different factors affected the number of viral particles on the bus. That included open versus closed windows, idling versus driving, and passengers with and without masks. Measures that impacted the safety of the driver were also considered.
The findings were encouraging. Simply opening bus windows reduced aerosol concentrations by 50 percent. Well-fitted masks were shown to limit the spread of particles from riders to just a few inches. And closing off the HVAC vent near the driver dramatically reduced their risk of infection.
You would be hard-pressed to find a face more closely linked to the fight against COVID-19 in the U.S. than Anthony Fauci, director of the National Institute of Allergy and Infectious Diseases. His resume, built over more than half a century of facing off against diseases like HIV/AIDS and Ebola, seems a perfect formula for creating a public health leader to deal with a pandemic.
And yet, repeatedly, the man and his message—wear masks, avoid enclosed gatherings with large numbers of people—have been attacked. During a podcast in June, he brushed aside the personal attacks, honing in on the mistrust of scientific guidelines.
“One of the problems we face in the U.S. is that, unfortunately, there is a combination of an anti-science bias that people—for reasons that sometimes are, you know, inconceivable and not understandable—they just don’t believe science…,” he said. “…And that’s unfortunate, you know, because science is truth.
“It’s amazing sometimes the denial there is. It’s the same thing that gets people who are anti-vaxxers, who don’t want people to get vaccinated, even though the data clearly indicate the safety of vaccines. That’s really a problem.”
Since COVID-19’s arrival in the U.S., politics, misinformation spread via social media and, in some cases, a handful of retracted research papers, combined to impact how well the public responded to new findings and directives.
Clack’s work during the pandemic has given him a front row seat to how well a variety of masks can prevent virus-sized particles from reaching the mouth and nose of wearers. To see people still refusing to wear masks and doubting their effectiveness, even well into the sixth month of the pandemic in the U.S., is troubling, if not surprising.
“If you want to just say ‘I’m tired,’ or ‘I just want to recover a little bit of the normalcy that I remember,’ I get that,” he said. “That’s a real thing, and there’s no reason to politicize that.
“But when you get to the point of not taking responsibility for your own COVID-19 fatigue, and you choose to justify your choices by saying the science is wrong, that’s where the problem is, because it’s immature. You’re in denial—willfully choosing to assign blame to someone else for a decision that is solely yours.”
There’s also resistance to the idea that understanding can change or grow as more information comes in.
“One of the things that I find frustrating is the notion that science has to be perfect, and perfect the first time,” said Cohn. “There’s almost like this game of gotcha with people saying, “I can find that you didn’t think this six months ago, therefore, why should I believe you now?”
Cohn also serves as associate director of the Center for Healthcare Engineering and Patient Safety, putting her in both the engineering and public health fields.
“Some people think that if you change what you say, like if ‘don’t wear a mask’ becomes ‘do wear a mask,’ that it’s not because you learned something new, it’s because your agenda has changed,” she said.
Clack’s expertise in dealing with airborne viruses resulted in plenty of calls from reporters during the pandemic. Even well-educated members of the media, he said, can be hesitant to allow science to evolve over time. One repeat interviewer asked in August why it had taken so long for researchers to focus on airborne transmission. Clack tried to explain.
“We always knew the disease could be transmitted by people breathing on each other,” he said. “Despite that, we spent March and April wiping down our mail and UPS packages because we thought, at that time, the primary mode was transmission by touch. That’s shifted now.”
Against that uneasy backdrop, U-M’s research teams pushed ahead. Not every project was a home run. Not every project went smoothly. But the proximity of medical/engineering personnel—with campuses located roughly one mile from each other—led to some exciting collaborations.
People’s unwillingness or inability to understand that science is an evolving process is a problem. And there’s also this perception that there’s an agenda.
“I think many hospitals or many engineering schools, on their own, could not have accomplished the degree of testing and evaluation that was done in a short period of time here at the University of Michigan,” said Keith Kaye, director of research at Michigan Medicine’s Division of Infectious Diseases, who worked with engineers on mask decontamination methods. “This is multi-discipline, collaborative work, and not just within the medical school or college of engineering separately, but between the two.
“It has really allowed us to do an incredible amount of diverse, high-level science in a very short period of time. That collaborative spirit, that strong fighting spirit and pride, at U-M has been impressive to me. It made me proud to work here.”
In a year unlike any other, with a seemingly endless supply of new obstacles and bad news, going back to the basics of research created something positive for many to hold on to.
“There have still been politics associated with this—there’s still turf and everything else,” said Cohn, based on her experience coordinating several of the projects. “But my sense of this is that we had a large number of people who basically said, ‘This is my family. This is my community, my planet. And this stuff matters more than anything I’ve ever faced before. And I’m going to bring whatever skills I have to the table to get it done.
“There was something incredibly comforting about feeling like, in the midst of this really frightening thing, I’m doing something about it.”