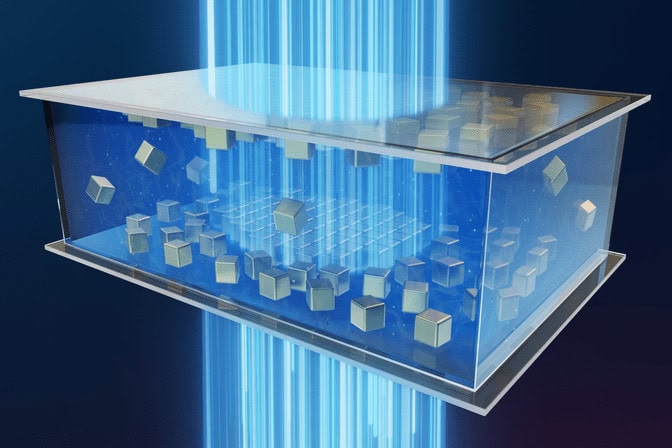
Morphable materials: Researchers coax nanoparticles to reconfigure themselves
It’s a step toward smart coatings that change color—or other properties—on the fly.
It’s a step toward smart coatings that change color—or other properties—on the fly.
Experts
A view into how nanoscale building blocks can rearrange into different organized structures on command is now possible with an approach that combines an electron microscope, a small sample holder with microscopic channels, and computer simulations, according to a new study by researchers at the University of Michigan and Indiana University.
The approach could eventually enable smart materials and coatings that can switch between different optical, mechanical and electronic properties.
“One of my favorite examples of this phenomenon in nature is in chameleons,” said Tobias Dwyer, U-M doctoral student in chemical engineering and co-first author of the study published in Nature Chemical Engineering. “Chameleons change color by altering the spacing between nanocrystals in their skin. The dream is to design a dynamic and multifunctional system that can be as good as some of the examples that we see in biology.”
The imaging technique lets researchers watch how nanoparticles react to changes in their environment in real time, offering an unprecedented window into their assembly behavior.
In the study, the Indiana team first suspended nanoparticles, a class of materials smaller than the average bacteria cell, in tiny channels of liquid on a microfluidic flow cell. This type of device allowed the researchers to flush different kinds of fluid into the cell on the fly while they viewed the mixture under their electron microscope. The researchers learned that the instrument gave the nanoparticles—which normally are attracted to each other—just enough electrostatic repulsion to push them apart and allow them to assemble into ordered arrangements.
The nanoparticles, which are cubes made of gold, either perfectly aligned their faces in a tidy cluster or formed a more messy arrangement. The final arrangement of the material depended on the properties of the liquid the blocks were suspended in, and flushing new liquids into the flow cell caused the nanoblocks to switch between the two arrangements.
The experiment was a proof of concept for how to steer nanoparticles into desired structures. Nanoparticles are too small to manually manipulate, but the approach could help engineers learn to reconfigure other nanoparticles by changing their environment.
“You might have been able to move the particles into new liquids before, but you wouldn’t have been able to watch how they respond to their new environment in real-time,” said Xingchen Ye, IU associate professor of chemistry who developed the experimental technique and is the study’s lead corresponding author.
“We can use this tool to image many types of nanoscale objects, like chains of molecules, viruses, lipids and composite particles. Pharmaceutical companies could use this technique to learn how viruses interact with cells in different conditions, which could impact drug development.”
Two side-by-side circles show a view of tiny squares clustered together in a messy arrangement, one in grayscale and another in a rainbow of colors. Their arrangement and colors change as different solvents are added to their environment When the solvent switches to a mixture of octane and butanol, the squares’ faces align more closely and the reds and yellows change to blue and green. The squares return to a messy arrangement with more red and yellow squares when they are put back in butanol. A scale next to the right shows how the squares’ color corresponds to how they have rotated: 0 and 90 degrees in blue, 22 degrees in aquamarine, around 40 degrees in yellow, and around 60 degrees in red.
An electron microscope isn’t necessary to activate the particles in practical morphable materials, the researchers said. Changes in light and pH could also serve that purpose.
But to extend the technique to different kinds of nanoparticles, the researchers will need to know how to change their liquids and microscope settings to arrange the particles. Computer simulations run by the U-M team open the door to that future work by identifying the forces that caused the particles to interact and assemble.
“We think we now have a good enough understanding of all the physics at play to predict what would happen if we use particles of a different shape or material,” said Tim Moore, U-M assistant research scientist of chemical engineering and co-first author of the study. He designed the computer simulations together with Dwyer and Sharon Glotzer, the Anthony C. Lembke Department Chair of Chemical Engineering at U-M and a corresponding author of the study.
“The combination of experiments and simulations is exciting because we now have a platform to design, predict, make and observe in real time new, morphable materials together with our IU partners,” said Glotzer, who is also the John Werner Cahn Distinguished University Professor and Stuart W. Churchill Collegiate Professor of Chemical Engineering.
The research is funded by the National Science Foundation.
Written in collaboration with Jaleesa Elliott, Indiana University Research Communications