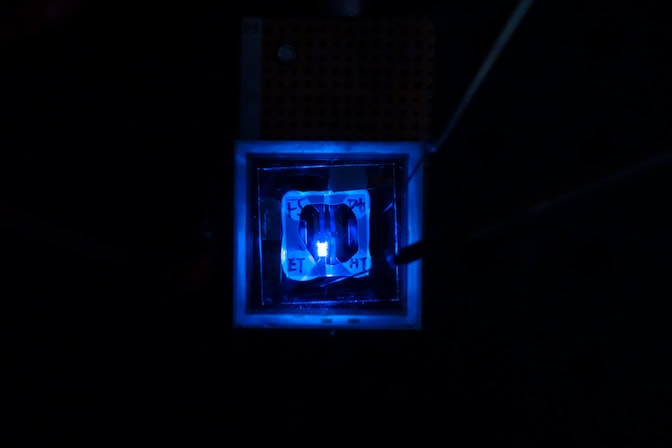
Efficiency upgrade for OLED screens: A route to blue PHOLED longevity
Commercial devices currently settle for less efficient blue OLEDs, but a set of design innovations has made an efficient blue that is as durable as efficient green OLEDs.
Commercial devices currently settle for less efficient blue OLEDs, but a set of design innovations has made an efficient blue that is as durable as efficient green OLEDs.
Experts
Blue phosphorescent OLEDs can now last as long as the green phosphorescent OLEDs already in devices, University of Michigan researchers have demonstrated, paving the way for further improving the energy efficiency of OLED screens.
“This moves the blues into the domain of green lifetimes,” said Stephen Forrest, the Peter A. Franken Distinguished University Professor of Electrical Engineering and corresponding author of the study in Nature Photonics.
“I can’t say the problem is completely solved—of course it’s not solved until it enters your display—but I think we’ve shown the path to a real solution that has been evading the community for two decades.”
OLED screens are standard in flagship smartphones and high-end televisions, providing high contrast and energy efficiency as variations in brightness are achieved by the light emitters rather than a liquid crystal layer over the top. However, not all OLEDs are equally energy efficient.
In current displays, red and green OLEDs produce light through the highly efficient phosphorescent route, whereas blue OLEDs still use fluorescence. This means while red and green OLEDs have a theoretical maximum of one photon for every electron running through the device, blue OLEDs cap out at a far lower efficiency.
The trouble is that blue light is the highest energy that an RGB device must produce: The molecules in blue phosphorescent OLEDs (PHOLEDs) need to handle higher energies than their red and green counterparts. Most of the energy leaves in the form of blue light, but when it is trapped, it can instead break down the color-producing molecules.
Previously, Forrest’s team discovered that there was a way to get that trapped energy out faster by including a coating on the negative electrode that helps the energy convert into blue light. Haonan Zhao, a recent Ph.D. graduate in physics, said it was like creating a fast lane.
“On a road that doesn’t have enough lanes, impatient drivers can crash into one another, cutting off all traffic—just like two excitons bumping into one another create a lot of hot energy that destroys the molecule,” said Zhao, first author of that study as well as the new one. “The plasmon exciton polariton is our optical design for an exciton fast lane.”
The details are based in quantum mechanics. When an electron comes in through the negative electrode, it creates what’s called an excited state in one of the molecules that produces blue light. That state is a negatively charged electron that jumps into a higher energy level and a positively charged “hole” that the electron leaves behind—together, they make an exciton.
Ideally, the electron would quickly jump back to its original state and fire off a blue photon, but excitons that use the phosphorescent route tend to hang around. Simply relaxing into their original state would violate a law of quantum mechanics. However, excitons very near the electrode produce photons faster because the shiny surface supports another quantum quasiparticle—surface plasmons. These are like ripples in the pond of electrons on the surface of the metal.
If the exciton in the light-emitting material is close enough to the electrode, it gets a little help with the conversion to blue light because it can dump its energy into a surface plasmon—a phenomenon known as the Purcell effect. It does this because the exciton oscillates a little like a broadcast antenna, which creates waves in the electrons in the electrode. This isn’t automatically helpful, though, as not all surface plasmons produce photons. To get the photon, the exciton must attach itself to the surface plasmon, producing a plasmon exciton polariton.
Forrest’s team encouraged this route by adding a thin layer of a carbon-based semiconductor onto the shiny electrode that encourages the exciton to transfer its energy and resonate in the right way. It also extends the effect deeper into the light-emitting material, so excitons further from the electrode can benefit.
The team reported on this last year, and they have since been putting this effect together with other approaches to finally produce a blue PHOLED that can last as long and burn as bright as a green one. These are the highlights of the design:
This study was supported in part by the Department of Energy and Universal Display Corporation.
Claire Arneson, a Ph.D. student in physics at U-M, also contributed to this study.
The device was built in the Lurie Nanofabrication Facility and studied at the Michigan Center for Materials Characterization.
The team has patented the technology with the assistance of U-M Innovation Partnerships and has licensed it to Universal Display Corp. Forrest and the University of Michigan have a financial interest in Universal Display Corp.
Forrest is also the Paul G. Goebel Professor of Engineering and a professor of electrical computer engineering, materials science and engineering, physics and applied physics.