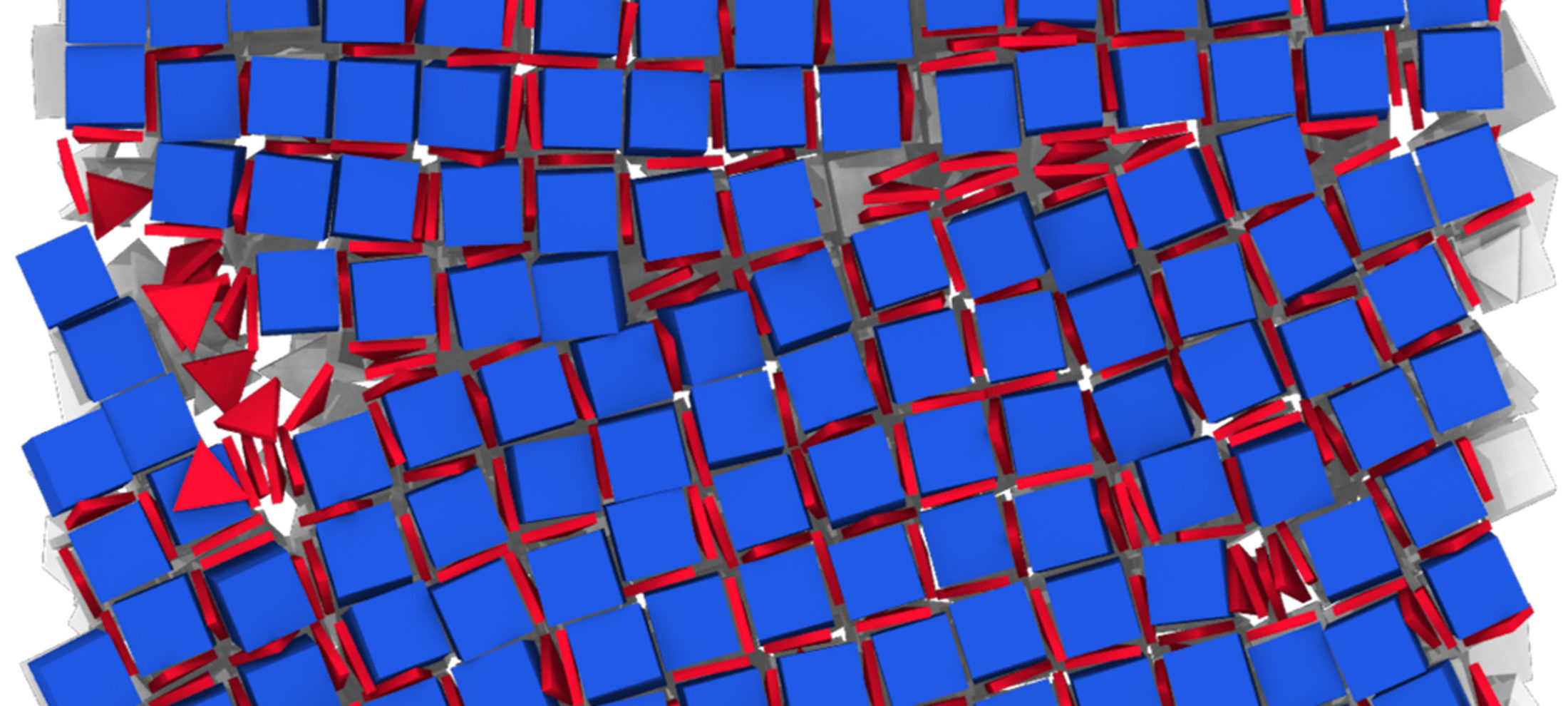
Nanoengineering integrates crystals that don’t usually get along
A team of computational and experimental engineers demonstrate a blueprint for building materials with new properties from nanocrystals .
A team of computational and experimental engineers demonstrate a blueprint for building materials with new properties from nanocrystals .
A blueprint for designing new materials using difficult combinations of nanocrystals has been developed by a team of researchers at the University of Pennsylvania and the University of Michigan.
The work could lead to improvements in nanocrystals already used in displays, medical imaging and diagnostics, and enable new materials with previously impossible properties.
Researchers can make materials with new and interesting properties by bringing together nanocrystals of different compositions, sizes and shapes. The challenge is doing that in an organized way. Now, the Penn and U-M team have developed a strategy that explores the available nanoparticles and figures out how to stick them together.
“It’s one of those problems where ‘like likes like,’” said recent Ph.D. graduate Katherine Elbert, who led this study while working in the lab of Chris Murray, a Penn Integrates Knowledge (PIK) Professor in materials science and engineering.
This tendency means that the different kinds of nanocrystals often separate themselves, forming disordered blobs rather than integrated, ordered solids.
“Here, we’re trying to overcome that barrier and make materials in which the nanocrystals are precisely coupled to their neighbors to hybridize their properties,” Elbert said.
Computer modeling by the group of Sharon Glotzer, the John W. Cahn Distinguished University Professor of Engineering at U-M, demonstrated a way to sidestep this barrier by coating the nanoparticles with molecules that alter its shape as far as neighboring nanoparticles are concerned.
“We can leverage those subtle changes to drive assembly as opposed to segregation,” said Thi Vo, U-M research fellow in chemical engineering.
One of the greatest challenges in the area of research is the sheer number and types of nanocrystals—with massive libraries of nanocrystals with varying chemical formulas, sizes and shapes.
“Putting each ‘brick’ exactly in the right place would be insurmountable,” Murray said. “But if you can find the rules by which nature wants to assemble nanocrystals, and you know how to optimize the conditions and the precise design of blocks, you now have that blueprint for making different classes of materials.”
Glotzer’s group combed through the library of particles that Murray’s group could make, modeling interactions between pairs of nanocrystals to see how they might assemble themselves into different desired structures. The computational study recommended sizes, shapes, material types and chemical environments for follow-up experiments in the lab.
The researchers focused on two classes of nanocrystals with vastly different compositions, sizes and structures in this study—one with interesting optical properties and the other with useful electrical properties. Usually, they don’t like to mix. But if they did, we could potentially combine them to make solar cells that can convert infrared light to electricity more efficiently, among other possibilities.
When the team precisely controlled the surface sizes and shapes of the nanocrystals with those coating molecules, so that the right combinations of crystals would attract one another, they were able to create integrated structures. These results can be applied to other types of materials with only minor adjustments.
“By building nanoscale components and organizing them under a universal set of conditions, we can get materials properties that don’t coexist or are exceedingly difficult to bring together. Now, we have a strategy to get the nanocrystals to couple and overlap,” Murray said.
The paper in Science Advances is titled, “Anisotropic nanocrystal shape and ligand design for co-assembly.”
This research was supported by a Multi University Research Initiative funded by the Office of Naval Research, with additional fellowship support provided by the National Science Foundation, Department of Defense, Air Force Office of Scientific Research, and the Vagelos Institute for Energy Science and Technology.
Murray is also the Richard Perry University Professor of Chemistry and Materials Science and Engineering and a professor of applied science. Glotzer is also the Anthony C. Lembke Department Chair of Chemical Engineering and Stuart W. Churchill Collegiate Professor of Chemical Engineering, and a professor of materials science and engineering, macromolecular science and engineering, physics and applied physics.