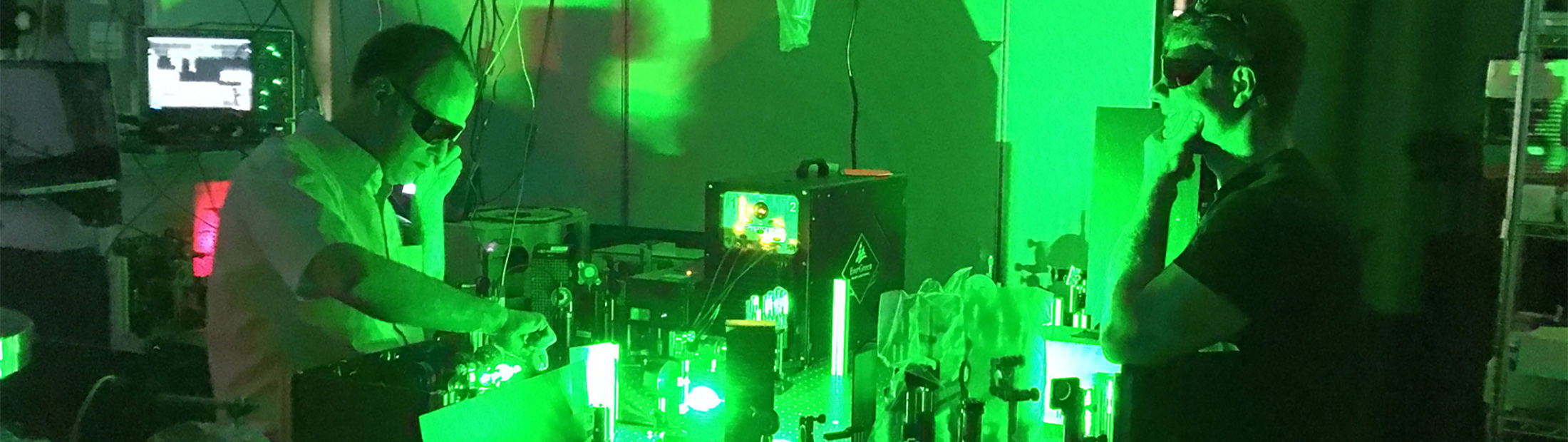
Using lasers to measure uranium enrichment
Nuclear energy and nuclear nonproliferation would both benefit from a faster, easier way to measure what proportion of uranium atoms can split.
Nuclear energy and nuclear nonproliferation would both benefit from a faster, easier way to measure what proportion of uranium atoms can split.
Identifying the purity and enrichment level of uranium is important both within the nuclear power industry and in nuclear nonproliferation. Those producing nuclear fuel need to know that the proportion of uranium atoms that can fission is at the correct level, usually between three and five percent. Those investigating nuclear facilities need to ensure that the uranium is not enriched above the level needed for power production.
Lasers could be the fastest way to make these measurements. Atoms and molecules can be identified by “fingerprints” that show up when they are probed with light. This fingerprint is essentially the structure of the “energy bands” that electrons occupy within an atom.
When an electron in an atom is given enough energy to jump into a higher energy band, it releases that energy as a photon with a particular wavelength when it jumps back down to a lower band. These photons can come in all colors of the rainbow and beyond, through infrared and ultraviolet light, and are known collectively as an element’s spectrum. Enough photons can reveal the band structure of the electrons in an atom, identifying what element it is. Distortions in the spectrum can identify whether that atom is part of a molecule.
The trouble with uranium is that its spectrum is very complex. With 92 electrons and about 1600 available energy bands, uranium’s “fingerprint” can contain upward of 100,000 different wavelengths of light, as Patrick Skrodzki, a PhD student in nuclear engineering and radiological sciences pointed out.
Skrodzki is a National Science Foundation Graduate Research Fellow working with Igor Jovanovic, a professor of nuclear engineering and radiological sciences, to identify the parts of uranium’s spectrum that can give information about enrichment levels. He told the Michigan Engineer about a study he recently published as a lead author, and which was chosen as the feature article in the journal Physics of Plasmas.
This technique is incredibly rapid since it is governed by rapid electronic processes that occur in atoms and molecules. In addition, the delivery of our laser excitation source and collection of the light from the plasma at long distances occurs at nature’s speed limit: the speed of light.
Currently, we are limited only by the speed of our electronics. Moreover, this method requires no sample preparation, and the entire system can be made portable and be done at a distance—which is advantageous when working with hazardous material such as uranium.
It’s an incredibly complex emission spectrum from which it’s difficult to identify and isolate a few useful fingerprints that can definitively say, “This is uranium.”
Then, if we do the experiment in air, the spectrum is even messier. The uranium tends to react with oxygen, and the resultant uranium oxides have even more complex, congested signatures, which further obscure the useful fingerprints in our signal.
But measuring in air is the most convenient way to test uranium—whether as quality control in the making of nuclear fuel or during an inspection to make sure that the uranium isn’t being enriched above levels you’d expect for nuclear energy. Hence, in our experiment, we studied the chemical pathways in the laser-produced uranium plasma, through which uranium reacts with oxygen.
Actually, yes. Uranium that is attached to oxygen can make it easier to tell the difference between isotopes of uranium—that is, the fissile uranium-235 and the more common uranium-238. The isotopic abundance of uranium can help us classify between natural, low-enriched (fuel-grade), and high-enriched (weapons-grade) uranium.
The kind of uranium atom that readily splits and releases energy—used in both nuclear fuel and nuclear weapons. Uranium-235 is the one we watch for. Uranium-238 is also relevant in this process, but the uranium-235 content is of far greater interest.
When uranium bonds with an oxygen atom, forming uranium monoxide, the bond between the uranium and oxygen atom can store energy. When it does, it vibrates. And that vibration, can tell you how heavy the uranium is. If it’s a little lighter, that’s uranium-235. Heavier, uranium-238. This shows up in the light emitted by the uranium oxide.
We shot a laser beam into uranium metal that was inside a gas-filled vessel. This way, we could vary the fraction of oxygen in a background gas of argon, an inert gas, in order to isolate reactions between uranium and oxygen. We observed the formation of uranium oxides via several non-invasive optical techniques including spectroscopy.
Yes.
All-optical techniques have certain advantages over more commonplace methods to detect uranium and measure its isotopic abundance. This is primarily because they provide rapid, portable measurement capability that can be used at a distance.
Consider the recent Joint Comprehensive Plan of Action, or Iran Deal, in which Iran agreed to limit its enrichment of uranium to a single facility and low-enriched/fuel-grade levels: Laser-based methods could be employed at the enrichment facility and any other inspected sites to quickly and safely verify the enrichment levels of uranium, judging compliance with the deal.
Laser-based spectroscopic techniques are incredibly robust and powerful. We can probe any form of target—solid, liquid or gas—and identify isotopes, atoms, ions and molecules regardless of the target composition. And, as previously mentioned, the techniques require no sample preparation, can be made portable, and are incredibly fast.
Currently, the Curiosity Rover on Mars employs laser-induced breakdown spectroscopy or LIBS (the same technique we use in the paper to study uranium) to study the composition of Martian rocks and soil. Although the library of known spectroscopic signatures is extensive and well-established for many elements and compounds, there remain some signatures which have yet to be identified or well-explored, such as those from higher uranium oxides (e.g. UO2, UO3, U2O2), at least in the visible region of the spectrum. In our work, we study one particular signature we believe to originate from uranium dioxide (UO2) which helps fill the gaps in that library.