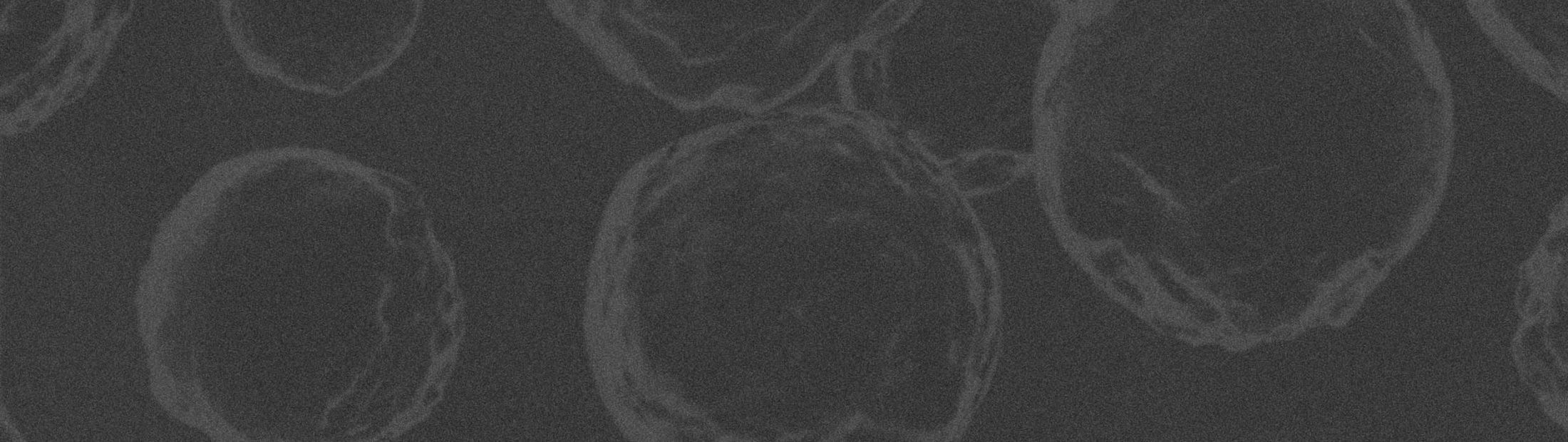
Are We Fighting Cancer Wrong?
Chemotherapy. Radiation. Surgery. Doctors go after the tumors that they can see.
Chemotherapy. Radiation. Surgery. Doctors go after the tumors that they can see.
Chemotherapy. Radiation. Surgery. Doctors go after the tumors that they can see. But the seeds that cause cancer to spread? Those are thought to be single cells or clusters of cells, traveling through the bloodstream. It’s hard to eradicate them with such blunt instruments.
Researchers call them metastatic cells, cancer stem cells or stem-like cancer cells. Not everyone is on board with what exactly these cells are.
“The controversial points include: Are cancer stem cells a fixed cell population, or is ‘stemness’ a state that any tumor cell can go through?” said Max Wicha, the Madeline and Sidney Forbes Professor of Oncology at U-M and a pioneer in cancer stem cell research.
He is working closely with engineers to answer this and other questions about cancer stem cells. Together, oncologists and engineers at Michigan are seeking to target these cells, shutting down cancer’s deadliest weapon: its ability to colonize other parts of the body.
Chemotherapy is a form of our usual go-to for exterminating unwanted colonists: we poison them. Antibiotics destroy bacteria without bothering our own cells because bacteria are very different from human cells. But cancer cells are human cells.
Cancer cells divide more often than normal cells, so conventional chemotherapy drugs go after particular stages in cell division. Cancer cells are more likely to be caught in these states. Still, the collateral damage can be devastating.
To make matters worse, stem-like cancer cells evade even this weak targeting because they don’t divide as often as typical cancer cells. They also seem to be tougher against radiation, with an enhanced ability to repair damage to their DNA.
Cancer stem cells’ resistance to treatment may help explain why cancer has a tendency to come back. The scans show that the tumor has shrunk, but the cells left behind may be the most likely to spread to other areas of the body, or metastasize.
And then there’s the immune system. Previously assumed to be a hapless bystander, now researchers believe that it is complicit in allowing cancer to spread (see Metastatic Decoy below).
Even so, oncologists at Michigan convey a sense of optimism. They have unprecedented tools at their disposal. Collaboration with engineers enables researchers to isolate and study cancer stem cells as well as the conditions that make a cell metastatic.
New devices are proving themselves in the clinic to inform patient care. One device offers a better measure of success for the clinical trial of a new breast cancer treatment than the usual yardstick of medical imaging.
Many of these researchers have come together to build a model for how metastasis occurs in humans — from an initial breast cancer tumor, through a simulated bloodstream, to a bone-like environment. The more they know about cancer and how it spreads, the better they will be at stopping it.
Cancer stem cells were first reported in 1994, in the highly regarded journal Nature. John Dick at the University of Toronto is credited with identifying them in leukemia, or cancer of blood cells. While ordinary leukemia cells divided frequently and were susceptible to chemotherapy, these other cells grew slowly, and his group noted that it was necessary to attack these cells specifically to avoid a relapse.
“Nobody really paid any attention to it, but we did because it seemed to me very logical, suggesting that cancer wasn’t just a disease of mutations but a disease of stem cells,” said Wicha.
Wicha teamed up with Sean Morrison and Michael Clarke, two professors of internal medicine and developmental biology at U-M, to run an experiment implanting human breast cancer cells into mice. They found that some cells formed tumors and others didn’t. For the cells capable of forming new tumors, they identified protein markers on the surfaces of the cells.
“And as soon as we published our study in 2003, within the next year, other groups started looking at stem cells in other kinds of cancer and found that virtually all cancers have these stem cells,” said Wicha.
Evidence is mounting behind the stem cell theory, but researchers are still tackling the question of whether stem cells are born or made. One of Wicha’s recent studies, published last June in the journal Lab on a Chip, showed that cancer cells rely in part on the cells around them for the signals that allow them to turn into new tumors.
…It seemed to me to be very logical, suggesting that cancer wasn’t just a disease of mutations but a disease of stem cells.
Euisik Yoon, a professor of electrical engineering and computer science, and his group led the work. They develop chips for capturing and growing single cancer cells. While computer chips are covered with elements like wires and transistors for manipulating electrons, microfluidic chips are networks of channels and chambers for manipulating cells in liquid samples.
This particular chip had a walled-in courtyard with narrow alleys leading to an area outside. The courtyard had nothing for a cell to cling to — just a slight depression to keep it in place — ensuring that only stem-like cells would survive there. They couldn’t leave, but they could exchange chemical signals with cells on the outside.
Beyond the walls, it was possible for cells to get a foothold, allowing non-stem cells to survive. Here, the researchers laid down a special breed of fibroblasts known to hang around with cancer cells. Fibroblasts are cells that build connective tissues, producing collagen, for instance. In control experiments, the isolated cancer cell had tumor cells for company or nothing at all.
The cancer cells surrounded by other cancer cells were only marginally more likely to divide and form a tumor, but those with fibroblasts were roughly three times as likely to do so. The fibroblasts seem to give off signals that encourage the cancer cells to form tumors.
“Cancer-associated fibroblasts boost cancer stemness,” said Yoon. “They also boost cancer drug resistance.” Stemness and drug resistance go hand-in-hand.
Decades of research with mice revealed much of what we know about cancer, but this method also obscured the effects of the signaling molecules produced by humans, such as those of the immune system. To see how cancer cells leave their initial tumor and strike out on their own, researchers build microfluidic chips that mimic blood vessels, organs and more.
But until the “Gizmo Club” of the early 2000s, oncologists at U-M didn’t have much contact with the people who design and build channel-filled chips (see Gizmo Club below). It was through these informal gatherings that Shuichi Takayama, a professor of biomedical engineering, connected with Gary Luker, an associate professor of radiology and biomedical engineering who studies metastasis. Together, they began use to Takayama’s microfluidic chips to determine how cells leave tumors and land elsewhere.
They identified signaling pathways — for instance, chemicals secreted by a fibroblast and picked up by a cancer cell — that encouraged cancer cells to get moving or to land in a blood vessel. These signals essentially lead the cells to become more metastatic. They showed that a variation on a single signaling molecule made a difference in how aggressively the cancer cells behaved. And that particular version wasn’t well targeted by the current drugs.
Around about 2002, Mark Burns, now the Anthony C. Lembke Department Chair of Chemical Engineering, and Daniel Hayes, the Stuart B. Padnos Professor of Breast Cancer Research, got to talking about how they could bring medical professionals and engineers together to find technological solutions to clinical problems.
The concept they piloted sent engineers down to the med school to hear presentations by doctors about problems they faced when trying to treat patients. Often, the problem would resonate with some of the engineers and then they would discuss potential solutions with the clinicians outside of the club.
“Bringing people together from different disciplines is so crucial in this day and age. Current problems are very complex, and it’s really hard for one person, no matter how intelligent they are, to understand all aspects of that problem,” said Burns. “At Michigan, you’re surrounded by so many other experts that you have this tremendous confidence they are bringing the same high-level knowledge and information to the table.”
Sometimes, these ideas turned into proposals, grants and results. Other times, they fizzled.
“If an idea came up, it was a tremendous effort to get a project going,” said Burns.
The missing piece, as Burns saw it, was seed funding to start a project immediately while excitement was high. The early results could then legitimize a proposal for a larger project funded by an agency like the National Institutes of Health.
In a sense, the Gizmo Club was a precursor to two initiatives at U-M. One is the Biointerfaces Institute, headed by Gizmo alum Joerg Lahann. The institute, started in 2012, holds workshops that bring in clinicians to discuss problems on a certain theme — such as capturing rare cells from a medical sample — with an audience of engineers. Discussions of potential engineering solutions are built into the program, and seed funding is available for the most promising ideas.
Or, if medical doctors and engineers come together some other way, they can still be funded through MCubed, an initiative that Burns co-launched in 2012. If three faculty from at least two different schools or colleges coalesce around an idea, they can test it out in a matter of weeks rather than months or years. Faster results mean faster progress.
The controversial points include: are cancer stem cells a fixed cell population, or is ‘stemness’ a state that any tumor cell can go through?
“New possibilities for anti-cancer drugs that target communication between tumor and non-tumor cells came into view,” said Takayama.
Engineers also develop ways to tell the cancer stem cells from the run-of-the-mill tumor cells. One of these, spearheaded by Yoon, is a chip that sorts the highly mobile cells from the laggards. When implanted in mice, the fleet-footed cells are much more likely to form tumors. This is the gold standard test for whether a tumor cell is a cancer stem cell, said Luker.
The researchers have been studying the highly mobile cells for genes that may make a cancer cell more aggressive. Luker’s lab will create cells that express these genes strongly — or not at all — and run them through Yoon’s chips to test how those genes affect cell mobility and their ability to grow into spheres that represent tumor precursors.
Devices like these — and there are many more — help explain the biology behind cancer stem cells and metastasis in a way that isn’t possible by looking at mice. But engineers aren’t just on the lab bench. They’re also working with clinicians.
Sunitha Nagrath, an associate professor of chemical engineering, focuses on catching cancer cells in blood samples, where they account for just one in about a billion cells. While not every cancer cell in the blood is a stem cell, this is how most stem cells are believed to arrive at metastasis sites.
Nagrath works closely with Wicha and other Michigan oncologists to design chips that can capture and handle cancer cells in different ways, for different purposes. One of her recent projects with Nithya Ramnath, an associate professor of medical oncology, studied whether circulating tumor cells captured from blood could monitor the progression of lung cancer as well as biopsies, which typically involves removing samples from the tumor with a large needle.
“We proposed an idea — a really bold idea — that we take cancer cells from these patients through blood samples,” said Nagrath. “We culture the cells, and we treat these cultures with the same drugs that she’s administering to the patients in the clinic.”
Capturing the cells gave Nagrath’s group the ability to find out which genes were active in the cancer cell, which tells Ramnath which drugs are likely to be effective. They saw the patient’s cancer develop a mutation that made it resistant to the first chemotherapy drug. Then, that mutation disappeared when they began targeting that mutation in the cancer.
This patient, a 42-year-old man, died after the cancer spread to his brain. Still, Nagrath is hopeful that her technology will help oncologists to monitor the mutations occurring in cancer more closely so that they can act quickly when drug resistance begins to occur. While biopsies pose a risk to the patient, blood draws could be done more frequently.
A variety of drugs are available to attack lung cancer’s various forms, but pancreatic cancer is not as well understood. Working with Diane Simeone, Lazar J. Greenfield Professor of Surgery, Nagrath is capturing cells from the blood of pancreatic cancer patients, looking for the genetic markers that make the disease more aggressive. These might also serve as targets for new drugs yet to be developed.
To make microfluidic techniques widely available at U-M, Nagrath is co-leading an effort to start a new service lab within the cancer center. It will enable more oncologists and researchers to capture and analyze cancer cells from blood samples.
One hot topic in managing the immune system’s role in cancer is whether drugs used for other immune-related diseases can be repurposed to fight cancer. Nagrath is working with Wicha on a clinical trial of a new drug combination to treat breast cancer — specifically targeting cancer stem cells.
“If you treat tumors with conventional chemotherapy, most cells die, so the treatment is monitored by watching for tumor shrinkage,” said Nagrath. “Cancer stem cell therapies are targeting only a few cells, so tumor size is not an indicator of successful therapy.”
Anne Schott, a professor of medical oncology, and Monika Burness, a lecturer in hematology and oncology, are currently running a clinical trial based on Wicha’s research showing that the arthritis drug tocilizumab can help treat an aggressive form of breast cancer, known as HER2 positive because the cells have HER2 receptors on their surfaces.
Two drugs attack via the HER2 receptors, killing most of the cancer stem cells, but Wicha’s work suggests that some stem cells are left behind. These have receptors for interleukin-6, an immune signaling molecule. Interleukin-6 is produced by our immune systems to help our bodies heal wounds, temporarily activating adult stem cells, but cancers keep this pathway open.
Tocilizumab binds to interleukin-6, preventing it from reaching the cells. Without exposure to interleukin-6, Wicha anticipates that the cancer stem cells will turn into non-stem cells, which can then be killed with the other two drugs.
Nagrath’s group will examine the number of cancer stem cells found in the patient’s blood samples over the course of treatment. If the treatment is effective, the numbers should fall.
Wicha isn’t the only oncologist at U-M looking into tocilizumab for fighting cancer. Jacques Nör, a professor in the school of dentistry who studies head and neck cancers, thinks it could slow or prevent metastasis in head and neck cancers.
Together with Yoon, he explored how to keep the interleukin-6 from encouraging cancer cells to migrate. A strong signaling pathway for interleukin-6 — meaning plenty of immune cells making the chemical as well as most cancer cells having receptors for it — is associated with high mortality in patients with head and neck cancers.
When interleukin-6 was removed from the system, either by preventing it from reaching the cells or ensuring that it was never there at all, cancer cells didn’t move as far. Tocilizumab may be a way to do that in patients. The study with Yoon’s system raised this possibility.
“This drug would have a chance to be eventually FDA approved for cancer, too, if the preclinical and clinical data show that it benefits the patient,” said Nör. “The next step is to work toward a clinical trial.
Cancer cells are believed to manipulate the immune system, paving the way for metastatic cells to receive a warm reception in the organ to be colonized. These sites send out chemical signals that are more concentrated at the metastatic site, like a homing beacon for an incoming cancer cell. Lonnie Shea, the William and Valerie Hall Chair of Biomedical Engineering, thought he could trick the cancer cells into landing elsewhere.
Shea and his group developed “sponges” that capture cancer cells from the blood stream when placed under the skin. Immune cells react to the sponge by rolling out the red carpet as far as a cancer cell is concerned, making the implant look even more inviting than an organ. The cancer cells found in the sponge are different from those that continue to circulate.
In experiments with mice, Shea and his colleagues found that the sponges reduced the number of metastatic cancer cells that landed in the liver by 64 percent; those in the brain were 75 percent fewer than in mice without the sponges. It’s an early detection system — it delays metastases and informs therapy decisions.
One of the questions on Shea’s mind is whether or not the cells captured in the sponges are cancer stem cells. To find out, he is working with Max Wicha, a U-M oncologist.
“The hypothesis is maybe we’re catching the worst cells and that’s why our animals are doing better,” said Shea. “An alternative hypothesis is the cancer cells being captured are not necessarily stem cells, yet the environment at the metastatic site turns them into stem cells.”
To help explore the question of how stemness evolves over the course of metastasis, many of these researchers are joining forces to make a system that can emulate the whole process.
“We’re basically trying to engineer the three key compartments that are required for metastasis,” said Luker. “We think the mechanics and signals in those environments control the ability of the cell to switch from one state to another.”
The compartments are the original tumor, the blood vessels, and the metastatic site. In their case, the initial tumor is a breast cancer tumor, and the site to be colonized is bone. The hypothesis is that regular tumor cells can be shifted to more stem-like states. Then, when they’re in the bloodstream, white blood cells called neutrophils spot that something is wrong and mount a suicidal attack, spewing a web of DNA and proteins at the offending cell.
The webs are armed to kill bacteria and yeast, but if left unchecked, they can damage human cells as well. However, evidence suggests that when cancer cells are exposed to these nets, they become more likely to form metastases. They are better at surviving in the bloodstream and invading new tissues.
When a cell arrives in the bone tissue, it is still in the stem-like state. One of Luker’s big questions is — can the cells be kept from dividing once they arrive?
“So you wouldn’t necessarily have to eliminate all cancer cells from a patient, but if you could make it essentially a chronic disease that you control, similar to HIV, you may be able to suppress the cancer effectively and permit the patient to have — if not a complete cure — a functional cure,” said Luker.
The model of the initial breast tumor and the bone will be made by Joerg Lahann, a professor of chemical engineering and director of the Biointerfaces Institute, and his group. He is well known for producing synthetic environments for growing stem cells that mimic conditions inside the body without relying on proteins and other materials harvested from animals. This keeps the cells from becoming contaminated with foreign proteins — or being influenced by them to behave differently than they would in a human.
“When I started talking to people like Max Wicha and Gary Luker and Diane Simeone, the issue is that when the cells that are collected through biopsy, especially those that are most tumorigenic, you just can’t grow them in a conventional petri dish,” said Lahann. But these cells grew well on his scaffolds.
To populate the initial tumors, Nagrath and Yoon will join forces to isolate cancer cells from patient blood samples as well as from mice. These can then be grown in Lahann’s scaffolds. Takayama and James Moon, a professor of pharmaceutical sciences and biomedical engineering, are handling the emulated bloodstream. This end-to-end model of metastasis should reveal more about how cancer spreads and provide a platform to test new ways to stop it.
If the last 60 years have taught us anything about fighting cancer, it is that there is very little reason to expect a single silver bullet. Cancers arise in many organs and cell types. The cells that make up cancers are themselves diverse.
Now, researchers are assembling a new toolkit against one of the most stubborn and complex killers of our time. Perhaps when oncologists learn how to target cancer’s aggressive cells, we may finally see more treatments that are effective at extending lives — without so much damage to quality of life.
Engineers are advancing health care. Interested?
Sign up to receive our upcoming email series about the intersection of engineering and health care. Our goal is to identify people who want to know more, and who may want to support the vital work happening at Michigan Engineering.