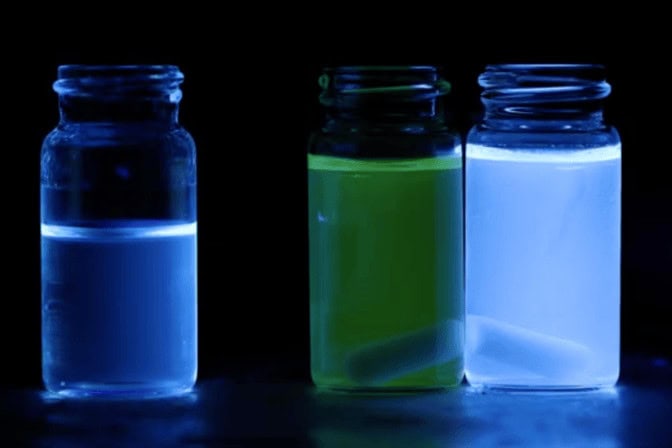
Faster organic phosphorescence for better display tech
Layering an organic material on top of 2D materials achieves stable, fast phosphorescent light emission without using expensive and hazardous heavy metals.
Layering an organic material on top of 2D materials achieves stable, fast phosphorescent light emission without using expensive and hazardous heavy metals.
Experts
Screens for TVs, smartphones or other displays could be made with a new kind of organic LED material developed by an international team, co-led by University of Michigan engineers. The material maintains sharp color and contrast while replacing the heavy metal with a new hybrid material.
Curiously, the material also seemed to break a quantum rule.
OLED devices currently on the market include heavy metal components like iridium and platinum, which improve the efficiency, brightness and color range of the screen. But they come with drawbacks—significantly higher cost, a shorter device lifetime and increased health and environmental hazards.
In OLEDs, light emission through the more energy-efficient phosphorescence is preferred over fluorescence, but phosphorescence happens more slowly, taking milliseconds or longer without the heavy metal component. Speeding up phosphorescence to happen in microseconds is necessary to keep up with modern displays, which operate at 120 frames per second, without producing a lingering “ghost” image. This is a key role of the heavy metals.
“We found a way to make a phosphorescent organic molecule that can emit light on the microsecond scale, without including heavy metals in the molecular framework,” said Jinsang Kim, U-M professor of materials science and engineering and co-corresponding author of the study published in Nature Communications.
0:00-0:17 – New organic molecule with fast phosphorescence material compared to conventional slow phosphorescence.
This first experiment demonstrates how the new organic material with ultrafast phosphorescent light emission (left) extinguishes immediately after the UV light is switched off, compared to conventional organic phosphorescent light emission (right) which dims slowly.
Phosphorescence is typically delayed because excited electrons must go through a conversion before emitting light on their way back down to the ground state. The new material layers an organic phosphor on top of a single-atom layer of molybdenum and sulfur (MoS2), which speeds up light emission by 1,000 times.
0:18-0:43 – New organic molecule creates green phosphorescence when combined with MoS2 and blue fluorescence on its own.
In the second experiment the new organic molecule contained in the vial on the left emits blue fluorescence under a UV light. When dispensed into the center vial, containing the semiconductor molybdenum disulfide (MoS2), the material emits green phosphorescence. When added to a vial containing water on the right, it continues to emit blue fluorescence.
Up to this point, fast phosphorescence was not possible without including a heavy metal, like iridium and platinum, within the molecular framework because they speed up the conversion excited electrons have to make before emitting light. This new material proves phosphorescence can be achieved without heavy metals, which can help develop cheaper and more environmentally friendly organic LED (OLED) screens for TVs, smartphones or other displays.
Dong Hyuk Park, professor of chemical and biomedical science and engineering at Inha University, and Sunkook Kim, professor of advanced materials science and engineering at Sungkyunkwan University, both in the Republic of Korea, are also co-corresponding authors.
The speed difference between fluorescence and phosphorescence is driven by what happens after electrons from the electrical current running through the OLED material slide into the high energy level within the molecule’s available electron orbitals, known as an excited state—sort of like jumping onto a rung of a ladder. In fluorescence, they can immediately release the energy as light, jumping back down to the ground state. But in phosphorescence, they have to make a conversion first.
The conversion has to do with the electron’s spin. Each electron has a partner in its ground state, and a quantum mechanical rule—Pauli Exclusion Principle—demands that they spin in opposite directions. But when an electron slides into that higher rung, it can end up spinning in either direction because each electron is now alone in its orbital. It only remains opposite its partner a quarter of the time, and this is the case that results in fluorescence.
Phosphorescence is three times more efficient because it harnesses the other 75% of excited electrons too, but it requires the electron to flip its spin before it can come back down. In conventional phosphorescent materials, the large atomic nucleus of the heavy metal generates a magnetic field that forces the same spin direction excited electron to turn quickly, resulting in faster light emission as it returns to its ground state.
The new material positions a 2D layer of molybdenum and sulfur near a similarly thin layer of the organic light emitting material, achieving the same effect by physical proximity without any chemical bonding. This hybrid construction sped up light emission by 1,000 times, achieving speeds fast enough for modern displays.
Light emission happens entirely within the organic material without having the weak metal-organic ligand bonding, helping the material last longer. Phosphorescent OLEDs that rely on heavy metals also use the metals to help produce the color, and the weaker chemical bonds between the metal and organic material can break apart when two excited electrons come into contact, dimming out the pixel.
Pixel burnout is a particular problem for high energy blue light that has yet to be solved, but the research team hopes their new design approach can help work towards stable, blue phosphorescent pixels. Current OLEDs use phosphorescent red and green pixels and fluorescent blue pixels, avoiding blue pixel burnout at the expense of lower energy efficiency.
Beyond the potential applications, analysis of this molecular hybrid system measured something once thought to be impossible—paired electrons sharing an orbital seemed to have a combined spin under dark conditions, suggesting a forbidden ‘triplet’ state when instead their spins should cancel one another out.
“We don’t yet fully understand what causes this triplet character in the ground state because this violates the Pauli Exclusion Principle. That is very impossible, but looking at the measurement data, yes, that seems to be the case,” Kim said. “That’s why we have a lot of questions about what really makes that happen.”
The research team will explore how the material achieves triplet character ground states while also pursuing potential spintronics device applications.
The team has applied for patent protection with the assistance of U-M Innovation Partnerships and is seeking partners to create devices using this new type of material.
This work was supported by the National Research Foundation of Korea grant funded by the Korea government and START grant from the U-M College of Engineering.
Collaborators from the University of California, Berkeley and Dongguk University contributed to the study. Jinsang Kim is also a director of academic programs for macromolecular science and engineering and a professor of chemistry.