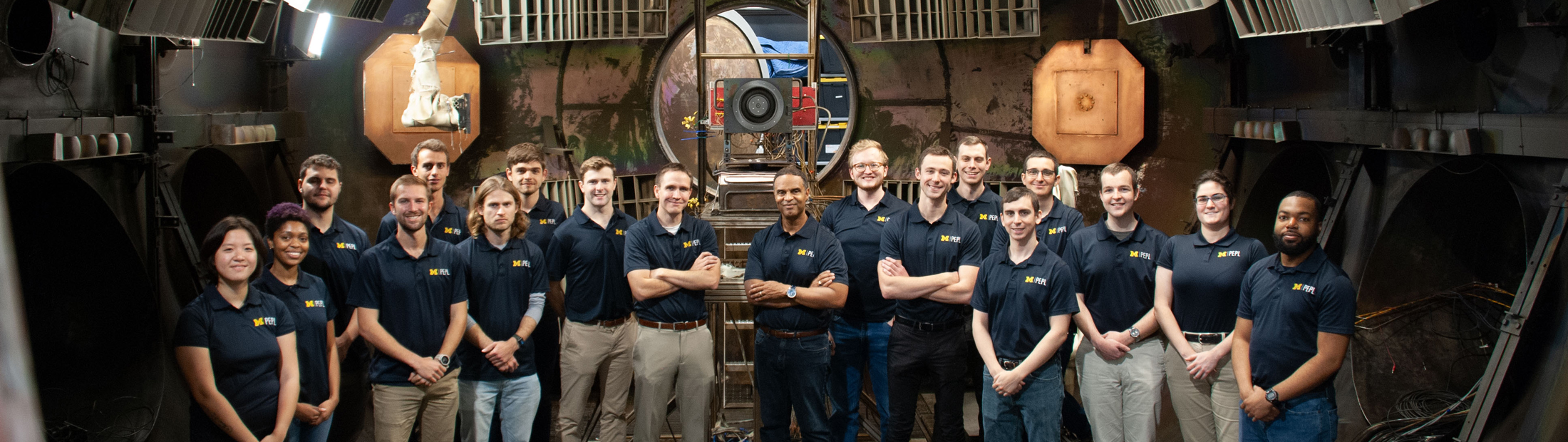
The storied history of a leading space propulsion lab
Alec Gallimore upcycled a lunar rover testing chamber into a world-class electric propulsion center.
Alec Gallimore upcycled a lunar rover testing chamber into a world-class electric propulsion center.
In December of 1984, U-M physics chair Larry Jones sent around a use-it-or-lose-it memo regarding a giant vacuum chamber that had been gifted to the University from the aerospace company Bendix. He and others whose work related to the physical sciences knew it was a treasure, but after years of disuse and maintenance costs of $1,500 per month (about $4,350 today), it was looking to the university leadership like a white elephant. It had once tested lunar rovers for the Apollo missions, but what could be done with it now?
The man who would make it into a lab that is now widely regarded as the best university facility for testing electric propulsion devices was, at the time, a junior at Rensselaer Polytechnic Institute. Luckily for him, the destruction of the lab was forestalled when Ronald Gilgenbach, director of the Plasma, Pulsed Power and Microwave Laboratory, proposed a project related to President Ronald Reagan’s Strategic Defense Initiative—also known as the “Star Wars” missile defense system.
When that fell through, Tom Adamson, then the chair of aerospace engineering at U-M, set his sights on bringing in someone who could use it for research in electric propulsion for space travel, which promised much higher performance than chemical rockets.
“It was a different time in hiring in academia,” Alec Gallimore, the outgoing Robert J. Vlasic Dean of Engineering, observed.
Adamson made a call to Gallimore’s advisor, Robert Jahn at Princeton, a professor of aerospace science who was leading one of the top labs in electric propulsion at the time, and asked for nominees among those graduating soon. It was still too early for Gallimore, who had another two years ahead of him. But one of the students suggested, Roger Myers (BSE Aero ’84), was a friend of Gallimore’s. Myers chose NASA, but later, he visited the U-M campus and toured the vacuum chambers. While he had no intention of moving into academia, he told Gallimore he had to apply. Myers told the aerospace faculty all about Gallimore too.
“It was clear to all of us that he was going to be a superstar, so the department did all that it could to recruit him immediately,” said Jim Driscoll, a professor of aerospace engineering who was on the faculty when Gallimore was hired.
As Gallimore recalls, he was never asked for his resume, but he visited U-M aerospace, giving a talk laying out his vision for research in electric propulsion, and seeing the cylindrical chamber that was cavernous as advertised—nearly 30 feet long with a 20 foot diameter. Even so, when he arrived at U-M, he didn’t intend to stay. The assistant professor role was going to be his work experience for his eventual application to become an astronaut.
Gallimore’s lab, called the Plasmadynamics and Electric Propulsion Laboratory (PEPL) started with plasma thrusters known in the Western Hemisphere, such as arcjets and the magnetoplasmadynamic thrusters that Gallimore had studied during his PhD. But since the fall of the Soviet Union in 1989, there had been talk in the field of electric propulsion technologies with efficiencies much better than an arcjet, the state-of-the-art for in-space electric propulsion at the time in the West, and ten times better than the chemical propulsion routinely used for space travel.
“Arcjets, these electric rocket engines, were sort of the talk of the town in terms of spacecraft propulsion for commercial spacecraft,” said Gallimore. “And then the Russians were bragging about their Hall thrusters, and which were much better in terms of performance, and so on,
to the point that people didn’t believe it.”
Representatives from the Ballistic Missile Defense Organization began visiting Russia. They brought those thrusters to US government labs, including NASA’s. Then, in 1993, Gallimore attended an electric propulsion conference and heard a presentation confirming that the wonderful things said about the technology were true.
“And when I came back from that conference I had a meeting with my research group, and I said, ‘Boys and girls, we’re now in the Hall thruster business,’” he recounted. “We became the first U.S. university to adopt Russian Hall thruster technology.”
To make this work, Gallimore needed to do three things. One: he had to upgrade the chamber so that it could reach a higher vacuum and also get rid of the propellant that Hall thrusters would emit more quickly. Two: he needed to fit it with better diagnostic equipment that would let his team see how the plasma behaved. And three: he had to get a Russian Hall thruster.
He submitted for a grant that he thought would enable him to do all three, but then, it turned out that the price verbally quoted to him over the phone by a U.S.-Russia joint venture formed to sell the Fakel SPT-70 (a 70-mm-wide stationary plasma thruster) was considerably lower than the quote that arrived on paper just when the proposal had to be submitted. He submitted the proposal with the mismatched written quote—there was no time to change it—and was not awarded a grant.
Soon after that disappointing news, Gallimore received a call from the director of science and technology at the Ballistic Missile Defense Organization, congratulating him on an excellent proposal.
“I said, ‘I hate to tell you this, but it wasn’t funded,’” Gallimore recounted. “He goes, ‘What do you mean it wasn’t funded?’”
The caller hung up abruptly and phoned NASA. About an hour later, NASA Glenn was offering $60,000—much less than the proposal requested, but enough to get started.
As for the thruster, a collaborator at the Moscow Aviation Institute (MAI) was able to provide a laboratory model of the same size as Fakel’s thruster. Within a few months, Gallimore picked up Sergey Khartov, deputy dean of international relations at the MAI, at the Detroit airport.
“I said, ‘Sergey, where’s the thruster?’ – thinking it would be in some fancy box. He said, ‘No problem,’” Gallimore recounted. “He opens up his luggage, moves around his socks and his pajamas, and there—in little zip lock bags—is the thruster and the cathode.”
Gallimore put together a new proposal for a grant from the Air Force Office of Scientific Research (AFOSR) with another young professor, Brian Gilchrist, in electrical engineering and computer science as well as the department that became the Climate and Space Sciences and Engineering.
“Alec was always seeking new and innovative ways to measure the behavior of electric propulsion thrusters to better understand their fundamental physics and improve their performance,” said Gilchrist. “He also wanted to understand how these thrusters interacted with other satellite subsystems.”
Still annoyed about the price jump that helped sink his original research plan, Gallimore was minded to call that “bootleg” thruster an SPT-70 in his research papers—the same name that Fakel was using. Gallimore compares the naming convention to a type of car, like a generic “coupe” rather than a branded “Mustang,” so Fakel couldn’t really stop him.
Still, the company wasn’t happy about it—their product would be mixed up with the laboratory model, which wasn’t going to touch the performance of their flight model. But with the Air Force grant, Gallimore could now promise test results that Fakel could use, if he could get that thruster into his lab. Fakel agreed, handing over a larger SPT-100. At $600,000 in 1995, that thruster was worth more than his grant.
Funding and thruster in hand, he and Gilchrist led a team exploring how the exhaust from the thruster would affect the solar panels and communication hardware on a satellite. Where should a Hall thruster be placed so that it could do its job without harming the spacecraft solar arrays or cutting off communication to and from Earth?
Around this time, Gallimore hung up his spacesuit dreams. His lab was picking up steam, he enjoyed teaching, and he found community with other faculty members. He reconsidered the move to Houston.
“The whole point I wanted to do was to go to Mars, not to go to Earth orbit,” said Gallimore. He’d planned the whole thing out in the ’80s.
“I thought, okay, I’ll jump into the astronaut corps in the ’90s. And, you know, I’d pay my dues being a shuttle astronaut, and then maybe 10 or 15 years later, I could vie for a mission to Mars. But it was clear by the mid-90s that we were not going to Mars in the first decade of the new millennium—and here we are now in the third decade,” he said.
If personally going to Mars wasn’t going to work out, he could at least continue working on the technology that might take some future generation to the red planet. The Hall thruster was particularly promising for this journey because of its efficiency. The initial acceleration is slower than with a chemical rocket, but electric propulsion offers top speeds that are significantly higher.
A Hall thruster works by accelerating a gas, often xenon or krypton, out the back, providing thrust in the forward direction. It relies on something called the Hall effect, which traps electrons in a ring. The propellant atoms run through that ring, colliding with the swirling electrons and losing their own electrons, becoming a plasma of charged ions and loose electrons. An electric field accelerates the ions, producing the thrust, while the electrons get pulled into the ring to keep the process going.
To better understand how these machines work, the PEPL team installed and developed technologies that would measure the electric and magnetic fields, the motion of the ions, the speed of the propellant, how charged the propellant ions were, and more. In the early years, they mostly tested thrusters developed by other researchers at NASA and in industry, including Russian institutes partnering with U.S. companies.
From the beginning, Gallimore set out to make PEPL a place where students felt valued and supported but also challenged to meet high expectations—and time and time again, those students succeeded. John Foster (PhD Appl Phys ’98), now a professor of nuclear engineering and radiological science at U-M, was one of his first students. He went on to work at NASA on the team that developed the NSTAR ion thruster, which flew on the Deep Space 1 and Dawn missions. In fact, the NSTAR came to Gallimore’s lab in 1998 for testing.
The NSTAR was followed by the larger and more powerful NEXT ion thruster. PEPL alumni who contributed to NASA’s ion thruster development program include Foster and Jon VanNoord (PhD Aero ’99), who is now a research scientist for the Space Physics Research Laboratory at U-M, and Dan Herman (PhD Aero ’05). Rohit Shastry (PhD Aero ’11) started testing NEXT in 2011 as a NASA engineer, then took the reins of its commercial iteration, NEXT-C, from 2016 until 2021. Foster and Van Noord discussed the project when NEXT-C flew on NASA’s asteroid redirect mission in 2021.
L. Brad King (PhD Aero ’98), Gallimore’s second PhD student, went on to start his own business building Hall thrusters for satellites, located in Houghton, Mich. Before coming to Gallimore’s lab, he’d been at NASA, looking for a facility where he could further research into electric propulsion through PhD studies.
“The best laboratory—and really one of the only laboratories for doing that—was at Michigan. And it was Alec’s lab that he was just bringing back online after decades in mothballs,” he said, referring to the old chamber that Gallimore was revamping into the Large Vacuum Test Facility, as it’s known today. “So right after graduating from Michigan with my undergraduate degree I headed right back to Ann Arbor to pursue my PhD.”
King went on to become a professor at Michigan Tech, continuing to explore the technology as it moved from an unfamiliar technology in the Western world to an in-demand means to control the orbits of the growing fleet of commercial satellites. When that shift became apparent, he was positioned to launch the startup Orbion Space Technology to provide those thrusters. Meanwhile, Foster has continued exploring electric propulsion but has also branched out into other applications for plasma, like water purification—including breaking down PFAS.
“Alec put our department ‘on the map’ by creating probably the best university research group in electric propulsion in the world,” said Driscoll. “Just after Alec joined us, we noticed that almost all of the very best grad students who applied to Aero wanted to work in his group. This trend has continued throughout his career. He has mentored an exceptional group of graduates who have had productive careers.”
In the next phase of Gallimore’s lab, starting around 1997, Gallimore and his students began building their own thrusters. At first, they ran on just a few kilowatts of power, like the thrusters they’d been testing.
“We were able to match the Russian performance right out of the box,” said Gallimore. “And my dear friend, Dr. Mitat Birkan, who was my AFOSR program officer at the time, told everybody that he would give me a D for that, if I were his student.”
To Birkan, matching existing capabilities wasn’t good enough—he wanted to see where the science was in Gallimore’s program. But Gallimore had always planned to take it further. Iain Boyd arrived at the University of Michigan as an associate professor of aerospace engineering, bringing his skills in computer simulation. They both wanted to find out what gave Hall thrusters that incredible efficiency and how to push to higher powers.
“In the computational modeling of Hall thrusters, we are still not able to predict thruster performance from first principles,” said Boyd, now a professor of aerospace engineering at the University of Boulder, Colorado. “This is therefore an area where detailed experimental measurements are essential to allow the models to make progress.”
It’s too complex to track the motion of the electrons inside Hall thrusters with computer modeling—the accurate simulations would take forever to run, and simplifications don’t reflect how the electrons move through the magnetic field. Instead, Boyd’s group used the data collected by Gallimore’s group to tell their models how the electrons behaved.
“The broad array of experimental diagnostics developed, implemented, and applied by Alec’s lab provided a treasure trove of data on Hall thrusters that allowed my research group to demonstrate that if we know how electrons move, we can indeed accurately predict thruster performance,” said Boyd.
The deeper understanding of how Hall thrusters function, discovered through simulations, helped Gallimore’s group produce more powerful thrusters.
Once the PEPL team outdid the Russian thrusters in terms of performance, they started looking at how to really push the power limits. The trade-off with a Hall thruster is that it runs best with a relatively low flux of propellant ions. Add more propellant, the thought was, and the performance would drop rapidly because the ring of electrons that ionized the propellant would be weakened from running into all that propellant. As a result, the propellant wouldn’t get as charged—and couldn’t be accelerated as much. The other problem is that if you turn up the power and propellant, the whole thing gets a lot hotter, and even the extreme cold of space can’t remove heat fast enough to keep the thruster from melting.
Gallimore’s team tried a variety of solutions to get more power out of the same footprint. They published and presented their work widely, and many of their ideas were picked up and incorporated into NASA and commercial satellites. During this phase, Mitchell Walker (PhD Aero ’05), now a professor of aerospace engineering and associate dean at Georgia Tech, and Kristina Lemmer (PhD Aero ’09), an associate professor of mechanical and aerospace engineering at Western Michigan University, graduated.
“Prospective students would see what the lab was like, smoke and sparks flying, everybody driving, trying to be world class. You’d work with everyone from everywhere, supporting each other, and Alec would support you. The people who wanted that environment joined,” said Walker.
Like many PEPL alumni, they still collaborate with the lab and with one another. Walker leads a major NASA-funded center to facilitate collaborations between NASA and universities that will help drive the transition to electric propulsion in space for human exploration beyond the moon. PEPL is part of the grant as well as alums including Lemmer, Foster and Boyd.
Lemmer has worked closely with the Michigan Space Grant Consortium from her time as an undergraduate at U-M onward, representing Central Michigan University as a professor for two years. The consortium links university students with opportunities to work on NASA projects. Gallimore served as its director for a decade, stepping down when he became dean. In addition to becoming research faculty and staff at universities, PEPL alumni have gone on to be engineers at NASA, program managers at funding agencies like the AFOSR, and entrepreneurs.
A lab as ambitious as PEPL might be expected to be cutthroat, but Gallimore remained attentive to the culture of the lab, offering his own support along with the expectation that lab members support one another.
“The culture in his lab was one of respect and friendship for your fellow lab mates,” said Lemmer. “From my experience, he ensured we all felt comfortable and valued as researchers. He provided us the freedom to make the mistakes we needed to learn, but was always there to guide us in the right direction.”
“He knew ‘world class’, and he would show you where that bar was,” said Walker.
In its second decade, the lab experimented with side-by-side arrays, two-stage acceleration and concentric rings. This latter design, known as a nested Hall thruster, worked particularly well. Into the 2010s, with funding from NASA as one of a few candidates for an electric thruster that could take astronauts to Mars, Gallimore’s team built a three-ring thruster, a meter across. In 2017, it set records for highest power for a Hall thruster, more than 100 kilowatts.
“And it’s already obsolete,” said Gallimore with a laugh.
While he didn’t drive that superseding innovation, it happened in the lab he built. Benjamin Jorns, an associate professor of aerospace engineering, took over much of the management of the lab when Gallimore stepped up to become dean in 2016. Gallimore had taken an interest in Jorns since he was a graduate student and had encouraged him to apply when PEPL needed a new co-director.
“I was very interested in the academic freedom that comes with running a university research program, and PEPL is one of the best academic institutions in the country with facilities to support the type of research I do,” said Jorns, who had been at NASA’s Jet Propulsion Laboratory.
Jorns led the team to reexamine the assumption about propellant and performance: did running at higher power, with more propellant, really send efficiency off a cliff? To find out, the team had to design a cooling system to keep the thruster from melting as they pushed it to five times its design power and nearly 10 times its design thrust. And they did, developing a thruster they called the H9 MUSCLE.
It wasn’t a straight line from idea to measurement, though. Leanne Su, a PhD student in aerospace engineering and lead author of the study, reports getting soaked repeatedly while they built the water cooling system. Then last October, a water pipe burst during a test, covering the thruster in ice that left rust when it melted. Many of the students thought the thruster was toast, but Jorns shares Gallimore’s level-headed style of leadership, offering optimism, support and high expectations.
“After the watery death of the H9 MUSCLE, we were kind of expecting to just call it, but he immediately pivoted to how we could recover and press on with the test. It ended up paying off big time,” said Su.
Under Jorns’s guidance, the team took it to pieces, cleaned everything and rebuilt it. In January of this year, they announced a result that overturned the assumption that Hall thruster performance took a nose-dive at high powers. They found they could get the same amount of thrust as the meter-wide X-3 but with one third the diameter and 10% of the 500 lb weight, opening exciting new possibilities for faster acceleration.
Now, as Gallimore leaves U-M to become provost and chief academic officer at Duke University, PEPL will stay right here, with Jorns as director. The vacuum chamber Gallimore helped save has now had a longer tenure as one of the best university facilities for plasma thrusters than it had for its original purpose of testing lunar rovers. Soon, it will also bear his name: the Alec D. Gallimore Large Vacuum Test Facility.
“The lab Dean Gallimore built has a world-wide reputation for cutting edge research in advanced propulsion,” said Jorns. “He has been a source of support, inspiration and guidance throughout my time here. My hope is to continue to build on this exceptional program he has created.”
Gallimore won’t be giving up the lab entirely though—he plans to stay in touch with Jorns and others and serve on PhD dissertation committees whenever students want the guidance of the man who founded it.
Gallimore is also the Richard F. and Eleanor A. Towner Professor of Engineering, an Arthur Thurnau Professor and a professor of aerospace engineering.