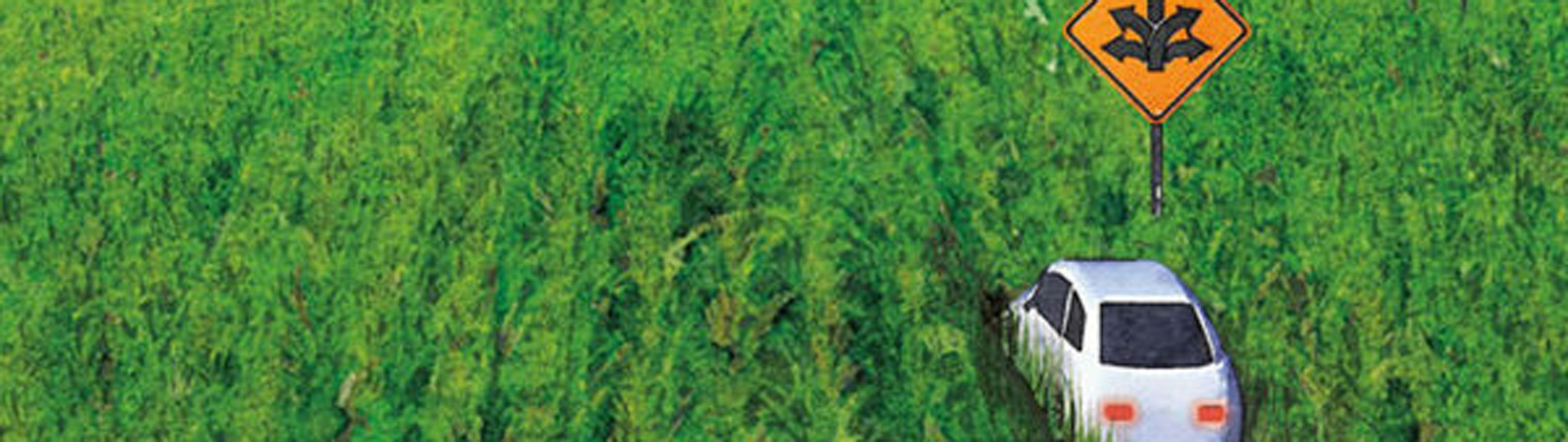
We’re doing ethanol wrong
Is there a way to get biofuels right?
Is there a way to get biofuels right?
It can damage small engines and older cars. It reduces gas mileage. It doesn’t store well. It competes with food. There are a lot of reasons not to like ethanol, at least the way we make and use it in the U.S.
And yet, pure gasoline is hardly available these days. We have wandered deep into the cornfield, and while many people have opinions about the best way out, we don’t have the will to leave. Where should we go? We could be using ethanol better, for one.
“I love the fuel,” said Margaret Wooldridge, an Arthur F Thurnau Professor and a professor of mechanical engineering, who argues that our current approach of blending with gasoline misses most of ethanol’s advantages. “There are a lot of caveats to criticisms of ethanol.”
Her line of research demonstrates that ethanol could result in lower emissions per mile than gasoline, for instance. Many others are exploring ways to make biofuel work better. But as for how we got stuck where we are? Well, we haven’t gotten past our starter solution for producing and distributing ethanol.
The federal government sets increasing targets for biofuels blended into gasoline and diesel sold at the pump. When the current policy, the Energy Independence and Security Act, was passed in 2007, it won support on both sides of the aisle. The Democrats liked the idea of carbon-neutral fuels displacing some of the gasoline in our tanks, and the Republicans liked the energy security of producing vehicle fuel on American soil.
It mandated the growth of an industry turning cornstarch into fuel, but it also capped how much that pathway could contribute to the renewable future at 15 billion gallons per year. We hit it in 2015.
But this was never viewed as the end goal for ethanol. By now, we were supposed to be making billions of gallons of “cellulosic” ethanol per year. Instead of using food sources, it is refined from fibrous plants like switchgrass that can be grown on poor farmland – or from agricultural “residues” like corn stalks and leaves. But so far, producing cellulosic ethanol typically costs more than $10 per gallon. In 2017, we made 10 million gallons of it anyway, which was still 4.99 billion gallons short of the target for the year.
As for foreign oil, corn ethanol has reduced our gasoline consumption by about 10 percent, but fracking has dwarfed its contribution to energy security, producing about 30 percent of our gasoline needs.
And some research suggests that biofuels are not living up to their climate promise. In 2016, John DeCicco, a research professor for the University of Michigan Energy Institute, published a paper in the journal Climatic Change arguing that biofuels, as produced today, are increasing carbon dioxide in the atmosphere.
In short, we’re getting neither the progress nor the returns we hoped for, and the policy hasn’t changed.
So, you pull carbon out of the air with plants, make fuel from the plants and put the carbon back into the air. How is that possibly worse than pulling carbon out of the ground and adding it to the air?
The idea that corn ethanol is inherently carbon neutral, so long as it doesn’t take too much energy to process into fuel, seems simple and obvious. This assumption is woven so deeply into the discussion of biofuels that many smart people have an easier time writing off DeCicco as an oil shill rather than accepting his thesis. His findings are squarely rejected by the biofuels community.
“I’m persona non grata around here,” said DeCicco with a wry half-smile, in his office at U-M’s Energy Institute.
His concept is equally simple and obvious. He takes a step back from the fuel carbon cycle to the global carbon cycle: We emit at a certain rate, and burning biofuels instead of petroleum doesn’t change that rate – at least the way we use them now. So for biofuels to be beneficial, an increase in harvest must compensate for all biofuel-related emissions.
DeCicco knew there were numbers available to do the math, but environmental groups and government agencies declined to fund his study. The American Petroleum Institute, however, has a financial interest in discovering that corn ethanol is not reducing our carbon emissions. So they gave DeCicco, a former senior fellow at the Environmental Defense Fund, money to explore whether biofuels could actually be causing more CO2 buildup in the atmosphere.
Oil money is considered dirty money in environmental circles, but he felt driven to look at agricultural data. He and his colleagues examined how much carbon was being fixed by crops, which is fairly straightforward to measure from the volume of the harvest. The paper assumes that stuff left behind on the field decomposes, sending carbon back into the atmosphere.
Biofuels, as produced today, are actually increasing carbon dioxide in the atmosphere. “If I were king, I would stop all biofuel production.”
John DeCicco
As a baseline, they assessed the total harvest of cropland in 2005 – dominated by corn and soy but also including wheat, alfalfa and cotton. For biofuels to put less carbon into the atmosphere, the increase in crop yields needs to make up for the emissions from fermenting the cornstarch into ethanol as well as the burning of the biofuel.
DeCicco’s analysis suggests that even as the carbon captured by harvests increased, the amount of carbon emitted through fermenting and burning ethanol was higher. His team calculated that, between 2005 and 2013, U.S. biofuel use had added 83 million tons of carbon to the atmosphere versus simply carrying on with burning fossil fuels.
That figure doesn’t include the “carbon debts” owed when carbon-rich natural lands – both forests and grasslands – are destroyed to provide extra cropland for growing biofuels in addition to food. Biofuel crops pay back these debts by displacing carbon that would have been emitted from fossil fuels, but it takes decades or centuries.
There are other factors beyond the scope of DeCicco’s analysis, such as changes in U.S. imports and exports of field crops, processing improvements that convert more cornstarch into fuel, and the possibility that no-till agriculture could fix carbon into the soil. Biofuels researchers argue that such limitations to the analysis produce a worst-case picture of the ethanol mandate’s early years rather than a representation of the potential for biofuels.
But DeCicco has support from climate change analysts who propose different land use solutions to atmospheric carbon, such as reforestation or reverting to grassland.
“If I were king, I would stop all biofuel production,” said DeCicco. “I wouldn’t zero out the research, but I would definitely zero out the mandates, the policies and the incentives to produce biofuels.”
Still, Wooldridge points out that this would leave farmers in the lurch, in an industry that already has tight margins and a high suicide rate. And even if we did try to transition away from cornstarch ethanol now, we’d be fracking more. We don’t have other scalable biofuel answers yet.
No easy carbon fix. Proponents of different carbon management techniques promote different statistics to make their cases, but each strategy has drawbacks. Forests can catch fire or go through periods when they emit more carbon than they fix because so much is stored above ground. Grasslands don’t always fix a lot of carbon even when they are irrigated or fertilized. And while corn’s numbers look good acre for acre, DeCicco argues that we haven’t been growing enough extra crops to fix the carbon emitted through producing and burning ethanol – we’ve just gotten by with less food.
Grassland source:
Conant, Richard et al. “Grassland management impacts on soil carbon stocks: a new synthesis,” Ecological Applications, 27(2), 2017, p. 665.
Forest sources: Schlesinger, W.H. and Emily Bernhardt. “Biogeochemistry: An analysis of global change,” Cambridge, MA: Academic Press, 2013, p.149.
Bellassen, Valentin and Sebastiaan Luyssaert. “Carbon sequestration: Managing forests in uncertain times,” Nature 506, 153–155 (13 February 2014).
Corn sources:
Low Carbon Fuel Standard Lookup Table Pathways, California Air Resources Board. https://ww3.arb.ca.gov/fuels/lcfs/fuelpathways/pathwaytable.htm.
Corn yield:
National Agricultural Statistics Service, United States Department of Agriculture. https://quickstats.nass.usda.gov/.
In 2007, the technologies to turn cellulose into fuel seemed to be right around the corner. Agricultural residues like corn stalks and leaves (known as corn stover) and non-food crops like switchgrass, poplar and hemp had been floated as the next great ethanol feedstock.
The trouble is, it still requires a lot of energy to turn cellulose into ethanol, no matter which feedstock you choose. As DeCicco observed, the commercially proven approaches to biofuels are merely variations on ancient themes: we use microbes to ferment sugars into ethanol, and we produce oils for biodiesel by squeezing them out of seeds.
“You can easily break starches down to sugars,” said DeCicco. “Cellulose is a sugar-based polymer that nature evolved to resist digestion.”
We know it’s possible to efficiently convert cellulose into chemical fuel – termites do it whenever they chow down on a two-by-four. But we haven’t been able to master it in a refinery.
“It’s a tough nut to crack,” said André Boehman, a professor of mechanical engineering and director of the W.E. Lay Automotive Laboratory, noting that U.S. cellulosic refineries are currently running at a demonstration level or shut down.
Current cellulosic ethanol plants rely on a pretreatment of the materials, such as corn stalks and straw, using heat and chemicals to begin breaking down the chains of sugars in the cellulose. They often produce electricity or pellets for stoves alongside the liquid biofuel, maximizing the efficiency of the energy conversion, but this production method remains pricey.
Meanwhile, scientists are still looking for ways to combine steps in the process, reducing the cost. Some nix the pretreatment by milling the plant material during the fermentation, but that requires hardy microbes.
Xiaoxia (Nina) Lin, an associate professor of chemical engineering, and her group used teams of microbes in a single reactor to break down corn stover to sugars and ferment them into isobutanol – another fuel that can replace gasoline in our current transportation infrastructure.
She experienced the fall in demand for biofuels when she and her team sought to launch a startup company based on the process in 2014. They ended up using the microbial team approach to turn other material from plants into a different molecule. Their new product would command higher prices if used in items like lotions, diapers and maxi pads.
“Biofuel, at that point in time, wasn’t an easy thing to do. For one thing, the oil price was really going down,” said Lin.
Advanced biofuel startups that were already rolling when oil prices tanked in 2015 made similar pivots or else went under.
“We need a rational way of valuing carbon,” said Boehman. “In some ways, it is unfair because we’re not paying for the damage that fossil fuels are causing, and we’re trying to make biofuels compete head-to-head.”
Beyond the making of the fuel, cellulosic feedstocks aren’t as straightforward as they once seemed either. Even though agricultural residues seem like cheap waste, they have value in preventing erosion and reducing the amount of fertilizer the field will need. And as for growing cellulosic crops, competition with food is only part of the consideration. We also have to weigh the cost of digging up natural carbon sinks.
Still, the cellulosic community has been exploring the cultivation-side problem. A review of cellulosic biofuel research, published in the journal Science in 2017, proposed that former farmers’ fields – abandoned due to low productivity – be planted with multiple native species, improving the diversity of plants. This approach would neither compete with food nor disrupt good carbon sinks.
We need a rational way of valuing carbon. “We’re not paying for the damage that fossil fuels are causing, and we’re trying to make biofuels compete head-to-head.”
André Boehman
They explored how much biomass could be grown on this “marginal land.” According to one study’s estimate, if we cultivated the most accessible of these fields for cellulosic ethanol, it could produce about 5.5 billion gallons. That’s a far cry from the 2022 goal of 16 billion gallons of cellulosic ethanol. However, G. Philip Robertson, a professor of ecosystem science at Michigan State University and lead author of the review, pointed out that with vehicle electrification and efficiency improvements, we might not need so much liquid fuel by 2050.
“Bottom line is that we could probably meet projected needs by using 50 to 75 percent of available marginal lands,” said Robertson.
If we develop better ways of making it, there are upsides to ethanol. But we’d have to use it differently. Mixing it into gasoline as a 10-percent-ethanol blend, known as E10, amplifies ethanol’s faults rather than its virtues.
Cars of model year 2001 or newer tolerate blends up to E15 (15 percent ethanol) well, but it can corrode metals and rubbers in engines that weren’t designed to handle its presence. This is because each ethanol molecule sports a troublesome oxygen atom. That oxygen (and its little hydrogen friend) can bind to metal or rubber, and it can break down lubricants.
Small and marine engines are most at risk of damage and running rough, according to reports commissioned by the Department of Energy. Small engines that aren’t optimized struggle with ethanol because it doesn’t need as much air to burn, Boehman explained. So when engines combine fuel with air, the mixture can be too lean on fuel. These are solvable problems – some newer small engines are already designed to handle E10 – but it will take time.
Ethanol-blended gasoline also doesn’t store as well because its oxygen atoms are magnets for the hydrogen atoms in water, forming somewhat casual chemical bonds known as hydrogen bonds. When ethanol absorbs water that has condensed inside a gas tank, for example, it creates a low octane gasoline resting atop a layer of ethanol and water. And that water can do some major damage if it gets into the engine.
Even in tolerant engines, ethanol has its critics. The big problem is energy density – a gallon of ethanol has only two-thirds the energy density of pure gasoline.
On the plus side for ethanol, its tricky oxygen molecules have already given us cleaner air by nearly eliminating soot in vehicle exhaust. But ethanol’s real problem, according to Wooldridge, is that we aren’t optimizing our engines to take advantage of it.
“Let’s imagine you put a donkey and a thoroughbred next to each other and have them pull the same wagon. Well, you only go as fast as the donkey,” said Wooldridge. And the donkey? That’s gasoline.
Pure ethanol, in an optimized engine, may not match gasoline in terms of miles per gallon. But it could outperform gas in miles per kilogram of carbon emissions. Ethanol is a high-octane fuel, which means it can be compressed much further than the typical gasoline ratio of 10:1. The more you can compress the fuel and air, the more efficiently your engine turns fuel into power.
The trouble is, if the compression ratio is too high for the fuel, the fuel starts combusting before the spark fires in the cylinder. That uncontrolled explosion, commonly known as engine knock, means that the piston gets shoved back, out of time with the other cylinders. Knock can wreck an engine.
We could be using ethanol to increase the octane number of our gasoline and make more efficient compression ratios standard. But we’re not doing that. Ethanol is blended with lower-grade gasoline to get E10 with the “regular” octane number of 87, Boehman explained.
Ethanol’s real problem is that we aren’t optimizing our engines to take advantage of it. “If a donkey and a thoroughbred pull the same wagon, you only go as fast as the donkey.”
Margaret Wooldridge
Even “flex fuel” vehicles that take E85 can’t take advantage of higher compression ratios because drivers can’t reliably get E85. They need to run on any blend from E10 to E85.
“If you had just E85 everywhere, I could give you compression ratios of 14:1 and 16:1,” said Wooldridge, almost wistfully. “But I can’t do that with a flex fuel.”
What she would like to see is a higher, reliable ethanol blend – that hasn’t been tainted with extra-low-octane gasoline – so that our engines could be designed for ethanol’s thoroughbred properties.
Of course, upping the standard octane requirements would reduce carbon emissions with or without the help of ethanol, since higher-octane fuels mean better compression ratios. This is a direction that environmentally-minded automotive engineers are also exploring.
With biofuel mandates continuing year to year, we seem to be stuck with the winner we chose, with little indication that we will improve how we use it. Nonetheless, the continuing research could eventually lead us to a better solution for the future of transportation energy.
This includes a host of biofuels, among which cellulosic ethanol and biodiesel from algae are perhaps the most discussed. Electric vehicles play heavily into projected fuel needs by the year 2050. And then there are options that would mean even bigger changes to infrastructure, such as hydrogen fuel cells.
We can wait for these technologies to compete with fossil fuels, or we can throw a finger on the scale with carbon taxes or carbon incentives that would hasten the market’s selection. Oil production will become more costly as oil becomes harder to extract, a trend temporarily stalled by the fracking of shale fields. And as the price of oil rises, Wooldridge and Boehman pointed out, a lot of new technologies become economically viable.
The question is, how much will the climate change in the meantime?
As DeCicco experienced, science is not a wholly objective pursuit – ideas float in and out of social and political favor, and that impacts what gets funded. Even so, two options stand out for our transportation future.
If we were going to choose a new winner right now, from the perspective of reducing reliance on oil and slowing climate change, hybrids should probably be the pick. By combining a rechargeable battery and electric motor with a smaller internal combustion engine, they cut gasoline consumption by roughly 30 percent. And even with the low gas prices of today, many are cost-effective over a 10-year vehicle life expectancy.
If hybrid powertrains were standard, we could reduce our gasoline consumption from 143 billion gallons to something in the ballpark of 108 billion gallons. And we could reduce it even further by designing the engines for high octane fuel, trimming off another 10 percent or so.
“Why not do both?” asked Boehman. “Why not have a really efficient internal combustion engine as part of a hybrid powertrain?”
Of course, manufacturing the batteries to take hybrids from 2 percent of U.S. cars sold to 100 percent is not a small problem. Wooldridge is in favor of hybrid vehicles, but she is concerned about making and discarding huge quantities of automotive batteries. She’s particularly worried about mining in countries that don’t have strong labor and environmental laws.
“People need to convince me that consuming hundreds of thousands of batteries doesn’t have social justice implications that could be worse for vulnerable communities,” said Wooldridge. “You may be trading in CO2 emissions for a heavy metal problem you get five, 10 years down the road.”
This is one of Wooldridge’s reasons for skepticism about fully electric vehicles, whose batteries typically outweigh batteries in standard hybrids by 10 times or more.
But others are looking into that battery problem. Anna Stefanopoulou, the new director of the Energy Institute and the William Clay Ford Professor of Manufacturing, is leading a project exploring how to make vehicle batteries sustainable. The actual recycling process is straightforward but expensive. The battery is discharged entirely to get all metals – the expensive metals as well as the cheaper lithium – on the cathode side for recovery. The costly part is separating the metals.
“The effort now is, can we rejuvenate the battery electrodes with an intermediate process so that we don’t have to go all the way back to the pristine metals?” said Stefanopoulou.
Plus, she said that electric vehicle batteries still have about 80 percent of their capacity when they are retired from cars. Could they have a second life on the grid, storing energy from a wind farm, for instance?
Of course, even if vehicle electrification works out, we’ll still need liquid fuels for flight and long-haul trucking.
“You’re never going to fly a jet on batteries,” said Bradley Cardinale, a professor in U-M’s School of Environment and Sustainability, who experiments with growing algae for biofuels.
Biodiesel is part of the Renewable Fuel Standard, but like corn ethanol, it was capped. While U.S. biodiesel relies mainly on soybeans, diverting more of the U.S. oilseed crop to biodiesel may speed deforestation in southeast Asia. It’s a matter of supply and demand: New palm oil plantations could make up the global shortfall in vegetable oils. In terms of climate change, the carbon debts for expanding biodiesel are high.
A different feedstock could change the game. That feedstock, many argue, is algae. It can be grown in the desert, where it doesn’t displace farmland, grassland or forest. Although most of the algal biofuel startups from the recent period of high gas prices have folded, researchers are still working to make the technology competitive with the current low prices. One such effort is a “cradle to grave” study led by Boehman.
“This’ll be one of the first times that we go all the way from growing algae in a pond to making biocrude, to upgrading that biocrude into fuel, and then testing it in an actual engine,” said Cardinale.
The challenge of growing algae is that it is prone to sudden die-offs. To get a continuous supply, Cardinale has focused on finding combinations of algal species that can – as a community – withstand pests, pathogens, and changes in sunlight and temperature. This is a departure from earlier efforts to develop “super-algae” that punched above their weight in oil production. These didn’t survive well outdoors.
Cardinale’s team saw success growing their algae communities in cattle tanks at the Edwin S. George Reserve, about 20 miles northwest of Ann Arbor, and in the new study, they will take their species mix and cultivation methods to the big leagues in Arizona, where future algae farms are expected to be sited.
Their algae crops will then be handed off to Phillip Savage, chair of chemical engineering at the Pennsylvania State University. Savage spent two decades at U-M, where he built a reputation advancing a technique called hydrothermal liquefaction. Unlike methods that simply extract oils from algae, his team heated the algae in water, which turns non-fatty parts of the algae into biocrude as well. Savage’s group can convert up to 70 percent of the energy in the algae to biocrude this way.
The biocrude, contaminated with more oxygen and nitrogen than engines like, will be cleaned up by the team of Levi Thompson, the Richard E. Balzhiser Collegiate Professor Emeritus of Chemical Engineering at U-M and now dean of engineering at the University of Delaware. In the final step, Boehman will conduct tests to help reveal which algae combinations provide better power and lower emissions.