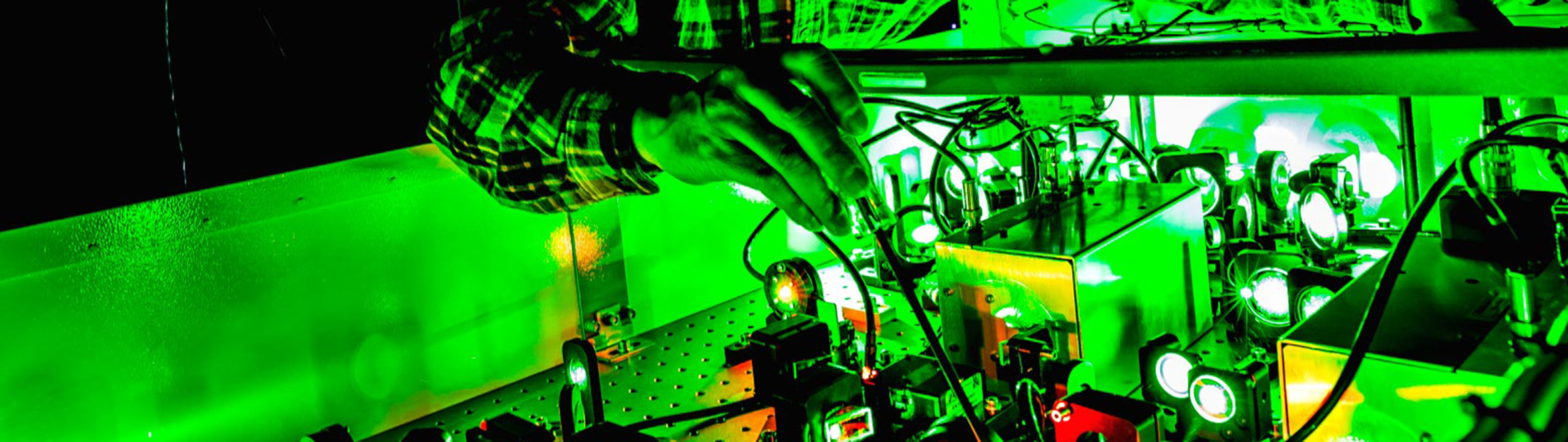
Precise pulses explore light’s magnetism
A new laser will investigate an unusual magnetic effect that may lead to efficient solar energy harvesting.
A new laser will investigate an unusual magnetic effect that may lead to efficient solar energy harvesting.
A new laser laboratory at U-M brings a unique capability to the state of Michigan and will help explore exotic interactions between light and matter. Phenomena to be studied at the $1.7 million facility, funded by the Air Force and U-M, could one day result in a new method for harvesting solar energy.
The Carrier-Envelope Phase laser facility is capable of creating very short pulses of light, just a few quadrillionths of a second long, and shaping peaks inside the pulse – something no other lab in Michigan can do currently. Stephen Rand, a professor of electrical engineering and computer science, designed the facility to investigate a surprising interaction his group first observed in 2007 and develop applications for it.
Light is composed of electric and magnetic wave components, oscillating at right angles to one another. Rand and his colleagues found that electrical insulators like glass could respond powerfully to the magnetic component of light waves.
Before the first U-M experiments, it was widely assumed that the magnetic component of light was too weak to matter for applications. Yet if the intensity is right, light produces magnetic effects that are more than a million times stronger than expected.
“The mechanism we’ve outlined takes place on each and every atom or molecule in the sample. And no one had reported these magneto-electric effects at the molecular level before,” said Rand.
The light waves manipulate the electrons on each molecule, creating regions of positive and negative charge. An electrical component, called a capacitor, stores energy in the form of charge separations like this. Because the light essentially turns molecules into capacitors, this strange interaction may offer a more efficient way to turn light energy directly into electrical energy.
No one had reported these magneto-electric effects at the molecular level before.
Steve Rand
Capacitors aren’t ideal for powering circuits because the voltage they provide drops as the energy is consumed. Even so, Rand anticipates that there is a way to convert the voltage in the material to stored energy in a battery.
This could change the game in solar harvesting because materials like glass can theoretically store 95 percent of the light energy in the form of a charge separation on their molecules, over a limited color range. In a normal solar cell, light hits a semiconducting material, which frees charges – electrons or positively-charged “holes” – that go on to power an electronic circuit. But freeing the charges typically uses only a small amount of the light’s energy – the rest of the energy is converted to heat, which is wasted.
“For solar cells, the conversion of sunlight to electricity is less than 50 percent,” said Rand. “We hope this process will eventually do much better.”
With the new laser, Rand and his colleagues will discover how exactly materials produce the charge separation and how it varies over time, crucial information needed to build a system that can harvest solar energy and store it in a useful way.
In most solids, light takes its orders from the medium it is passing through. However, to get the strong magnetic interaction, Rand and his group have shown that in this case, light alters the material in an important way as it moves through it.
“Until now, people have thought the only way to induce significant magnetic response at the frequency of visible light was to carefully engineer the structure of the medium,” said Rand.
But rather than decorating the material with metals or shaping the surface, his group observed magnetic effects in uniform materials like glass, known broadly as dielectrics. Dielectrics are electrical insulators that can support charge separations on their atoms or molecules but do not conduct electric currents. This makes them candidates for storing electrical energy.
In the magneto-electric interactions to be studied at the new facility, the electric field of the light sets the electrons attached to the molecules in the dielectric into motion. The positive holes and negative electrons follow straight lines at first, running to opposite sides of the molecule as the light’s electric field swings from positive to negative and back. Because this charge separation averages out to zero, it is not feasible to harvest the energy.
However the charges are also subject to the magnetic field of the light, which bends the line into an arc. Curved motion produces small circular currents, which essentially magnetize the dielectric. This magnetic force always pushes charges in the same direction, along the light’s path, even when the light’s electric field reverses. This produces a static charge separation, and that stored energy can potentially be harvested.
With their previous laser, Rand’s team could not control the timing of the peak electric or magnetic forces from one pulse to another. This meant they couldn’t control whether any pulse would build on the motion driven by the previous pulse or put on the brakes.
With the new laser, they can control when the electric and magnetic fields reach their peaks, so they can design a train of pulses that will build up the maximum charge separation effect. Alternatively, they can design pulses to turn the effect on and off, proving that the mechanism is understood.
In early experiments, the team will look at how different materials respond to different intensities of light. In order to harvest solar power, they will need to identify a material that has a strong charge separation effect in response to relatively low-intensity light.
Many unusual phenomena observed with lasers don’t happen with ordinary sunlight because they rely on properties of laser light such as the tight control of the wavelength or the alignment of the waves. However, these properties aren’t important for producing the charge separation. Regular sunlight, with its broad spectrum of wavelengths and the different timings of the waves, does just fine because all these waves still have magnetic components.
“This is an amazing aspect of optically-induced magnetic effects which is crucial for potential applications in energy conversion,” said Rand.
After the initial experiments exploring the effect in different materials, Tuan Trinh Ayesheshim Kebie and Krishnandu Makhal, research fellows in electrical and computer engineering, will study how magnetically-induced changes affect a second light pulse that crosses the initial beam inside the material. The frequency of the “probe” pulse is expected to double – something that it does not do ordinarily in dielectrics like glass.
Magnetism inside the material brought on by the main beam can also cause the electric and magnetic components of light to travel at different speeds, creating a twist in the orientation of the light field. Rand and his team anticipate that this could open the door to new communication devices that rely on light rather than electrons. Another research fellow in electrical and computer engineering, Hengky Chandrahalim, has been investigating induced magnetic effects in microscopic structures to see if this concept can work at ultralow power levels.
In addition to the experiments to understand the magnetic interaction, the new facility will provide outside researchers in Michigan the opportunity to work at the forefront of “shaped” laser pulses. For example, Marcos Dantus, a professor of chemistry at Michigan State University, is developing a system that could be used with the new laser to produce so-called “square” pulses that go from “off” to “on” very abruptly. Such pulses could measure extremely fast processes such as light-induced chemical reactions that evolve rapidly in time, providing unique measurement capabilities at the new facility.
The new laser facility is housed in the Center for Dynamic Magneto-Optics (DYNAMO).