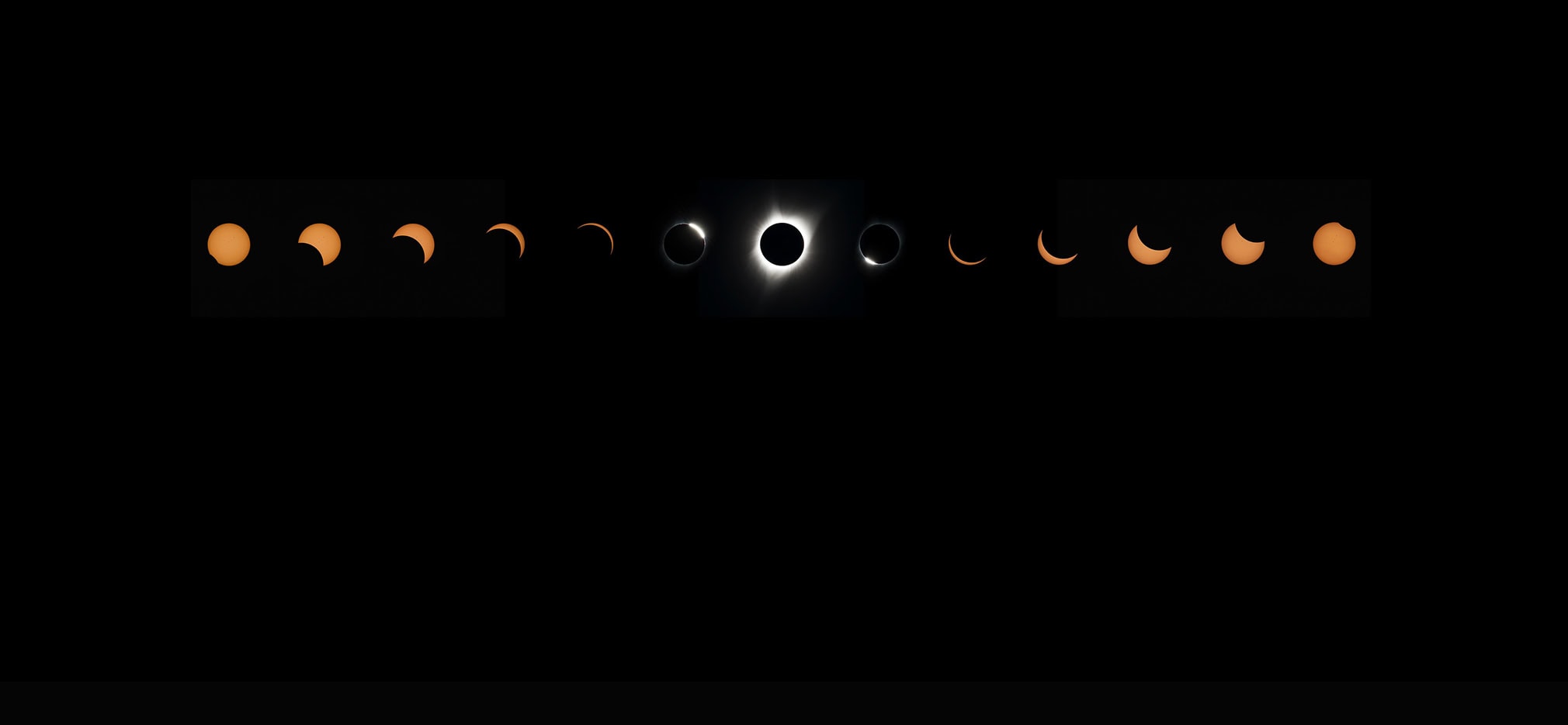
Seeing the sun in a new light
For scientists, students and the rest of us, the April 2024 eclipse helped illuminate some of the sun’s deepest mysteries.
PHOTO: NASA/Aubrey Gemignani
Published:
Updated:
Contents:
We have reached totality,” boomed the voice of an emcee over a loudspeaker as an eerie twilight fell over Indiana’s Taylor University campus at 3:09 p.m. on April 8, 2024. There was clapping, hooting and hollering from the audience of around 2,000 spectators, now standing in the moon shadow of a total solar eclipse.
The crowd reveled in the light show that had just appeared around the moon’s silhouette. White sunlight bounced off the rays of electrically conductive gas, or plasma, that stretched outward from the edge of the moon, forming a crown-like aura that inspired the latin name for the sun’s outer atmosphere—corona.
Nearby, hunched over laptops perched on folding tables, a group of University of Michigan undergraduates were watching something completely different: radio frequency data, in the form of red, yellow and green lines crawling across their screens. The students were part of the SunRISE Ground Radio Lab, a project led by Mojtaba Akhavan-Tafti, a U-M climate and space science and engineering associate research scientist, who studies the sun’s magnetic fields and their effects on Earth.
Starting in June 2023, SunRISE Ground Radio Lab provided students at 17 high schools across the United States with radio antennas. The students installed them at their schools and have been learning how to operate them ever since. They, along with the undergrads at Taylor, used them to watch for radio bursts during the eclipse, when the moon blocked the constant buzz of background noise that ordinarily comes from the sun.
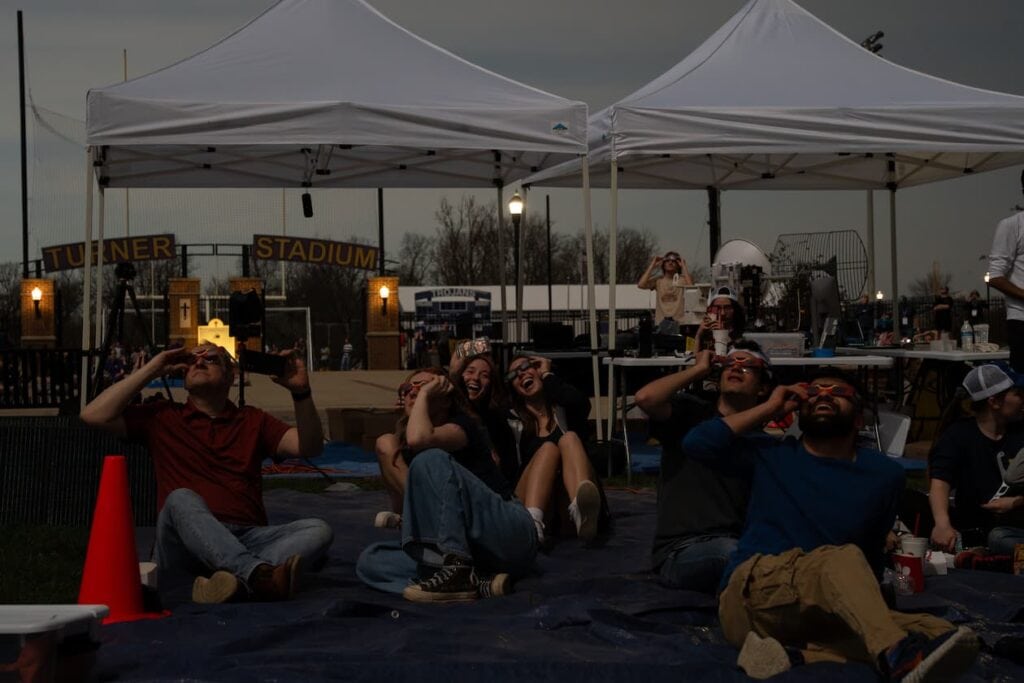
The eclipse was a high-water mark for NASA’s Heliophysics Big Year, a global celebration of solar science that takes place between October 2023 and December 2024. For U-M researchers who have dedicated their careers to building a better understanding of the sun, the eclipse was a rare opportunity to gather scientific data that’s normally overshadowed by the sun’s blinding light in the visible spectrum and the constant buzz of radio noise at longer electromagnetic wavelengths. It was also an opportunity to get budding young scientists like the SunRISE high school students involved in scientific research.
Working from classrooms, labs, airplanes and parking lots across the country, researchers, students and citizen scientists used the three-minute eclipse to fuel months of research and discovery aimed at shedding light on the sun’s most persistent mysteries.
How the sun flouts physics
The sun is made of layers of plasma. The hottest and densest plasma is at the sun’s core and the surrounding layers get progressively cooler and thinner, eventually becoming so thin that scientists consider the outer layers to be an atmosphere.
The corona, however, doesn’t follow the same rules as the rest of the sun. In fact, it doesn’t even follow the rules of basic physics. It’s nearly 200 times hotter than the sun’s surface despite being farther from the heat source at its core. The corona is so searing that its plasma moves fast enough to escape the sun’s gravity and stream away at more than 1 million miles per hour. This plasma sparks the impressive rays and loops that eclipse viewers saw from the ground. It then races across the solar system as the “solar wind,” dragging the sun’s magnetic fields along with it.
The solar wind underpins the flow of magnetic fields and charged particles throughout the solar system, which is known as “space weather.” Understanding space weather is more than a matter of curiosity; when it grows severe, it can disrupt our technological systems and harm astronauts who are in space during an event.

“
Forty minutes is just enough time to make some changes to the power grid to help prevent damage.
The most hazardous space weather happens when large clouds of plasma and magnetic fields called “coronal mass ejections” erupt from the sun, or when jets of highly energized charged particles called “solar energetic particles” accelerate toward Earth, following the sun’s magnetic fields like a kind of cosmic highway. When these solar storms arrive, they can deliver dangerous doses of radiation to astronauts, damage satellites and disrupt power grids on the ground.
One of the more disruptive coronal mass ejections in recent years left around 50,000 people without power in Sweden in 2003. The largest recorded storm caused by a coronal mass ejection, called the Carrington Event, occurred in 1859. It damaged telegraph systems and caused visible aurora in skies around the world. A similar event today would likely cause widespread blackouts and lasting damage to the power grid.
Advance warnings of hazardous space weather could help us prepare for and mitigate the damage, but scientists don’t have enough knowledge of the sun to predict a solar eruption before it happens. They can’t identify a hazardous coronal mass ejection until it’s about an hour away from impact on Earth, and the advance warning of a solar energetic particle event can be as little as 10 minutes. Even fundamental questions like how the corona’s plasma gets hot enough to accelerate into the solar wind are not fully understood.
“If we don’t know the answer to these questions, the rest of the field doesn’t have much of a real foundation to stand on,” Akhavan-Tafti said.
Protecting the grid during the May 2024 solar storm
Michigan Engineering has been a leader in tackling these heliophysics and space weather forecasting challenges for decades. U-M researchers lead the newly funded CLEAR Center, which NASA will rely on to predict the activity of solar energetic particles between the Earth, Mars and the moon.
In addition, U-M researchers have played lead roles in key solar missions, including NASA’s Parker Solar Probe and the Solar Orbiter, which is a cooperative mission between NASA and the European Space Agency. They also developed the Michigan Geospace Model, the only space weather model used by NOAA’s Space Weather Prediction Center to forecast the impact of geomagnetic storms on the ground based on measurements of the solar wind.
Part of NOAA’s toolkit since 2016, the Michigan Geospace Model helped the Prediction Center prevent disruption to the world’s power grid during the severe geomagnetic storm that hit Earth in May 2024.
By measuring when the storm’s coronal mass ejections flew past the Advanced Composition Explorer satellite, which is parked between Earth and the sun, it predicted the storm 40 minutes before it arrived. That gave the agency time to warn power companies of a severe geomagnetic event.
“Forty minutes is just enough time to make some changes to the power grid to help prevent damage,” said Daniel Welling, a U-M assistant professor of climate and space sciences and engineering. “A planned shutdown could be easier than doing nothing because if a transformer dies, you can’t turn the power grid back on until you compensate for all the damaged equipment. It’s easier to slowly ramp an intact power grid back online than repair a damaged one. The most sensitive parts are the high-voltage transformers, which are individually made to order, take many months to create and are very expensive.”
Power companies, including New Zealand’s Transpower, were able to make adjustments and keep the grid running. They shut down redundant and sensitive power lines and routed their power to more robust sections of the power grid. As a result, the 2024 geomagnetic event was far less disruptive than the 2003 storm, with no major disruptions to the world’s power system.
Close encounters with the sun
Launched in 2018, Parker Solar Probe has flown into the corona to uncover new information about how its plasma gets so much hotter than the surface of the sun. During Parker’s unprecedented journey, it measured the density and temperature of the corona’s plasma using instruments developed by Justin Kasper, a professor of climate and space sciences and engineering.
By pairing this data with magnetic field measurements made by other instruments on the probe, scientists have discovered that the sun’s magnetic field has thousands of S-shaped kinks near the corona. The stronger the magnetic field gets in these kinks, the faster the plasma flows. Some scientists think these magnetic zigzags, called “switchbacks,” could be moving magnetic energy to parts of the plasma where it otherwise wouldn’t be, potentially heating the solar wind in the process.
Parker’s measurement capabilities, however, are limited to its immediate vicinity. To get a wider vantage point, NASA and the European Space Agency launched Solar Orbiter on Feb. 9, 2020.
The orbiter is positioned farther from the sun than Parker, enabling it to photograph larger areas of plasma.
Solar Orbiter can also sample the solar wind using a sensor that was developed in part by Susan Lepri, a professor of climate and space sciences and engineering, and Jim Raines, an associate professor of climate and space sciences and engineering.
The sensor can detect coronal mass ejections traveling along the solar wind by identifying the heavy particles—like charged iron atoms—that accompany them.
By comparing the location of these heavy particles with photos of coronal mass ejections on the sun, scientists can trace where on the sun the particles likely came from, helping them understand how the storms form. That information is critical when building models to predict solar eruptions in advance.
The data collected by Parker and Solar Orbiter has enabled scientists to get a closer look at the sun than ever before, and the new CLEAR Center is combining the information gathered by these and other space missions into a benchmark public dataset for creating 24-hour forecasts of solar energetic particle storms.
“Because Parker Solar Probe and Solar Orbiter are viewing the sun in ways most space instruments haven’t, they are collecting data where we haven’t had much information before,” said Lulu Zhao, a research associate professor of climate and space sciences and engineering and the principal investigator at the CLEAR Center. “That can help us know whether our forecasting models are accurate for locations in the middle of the solar system, which builds confidence in our ability to forecast when solar energetic particles might hit space missions at the moon and Mars.”

New eyes on the sun
A constellation of satellites is helping scientists study the sun and the solar wind

Parker Solar Probe
DISTANCE FROM SUN
3.8 million miles during closest pass
SIZE
10 feet long and 3 feet in diameter
WEIGHT
1,510 pounds
FUNCTION
Parker will measure energetic particles, electric fields, magnetic fields, visible light and radio emissions within the solar wind.
PHOTO: APL/NASA GSFC

Solar Orbiter
DISTANCE FROM SUN
26 million miles during closest pass
SIZE
8 feet wide, 10 feet long and 8 feet deep
WEIGHT
3,800 pounds
FUNCTION
Solar Orbiter will be taking photos of the sun and eruptions from a polar orbiter for the first time and is measuring the chemical composition of the solar wind.
PHOTO: ESA/ATG medialab

SunRISE
DISTANCE FROM SUN
93 million miles
SIZE
A series of six satellites, each about the size of a cereal box.
FUNCTION
The six SunRISE satellites will be spaced around six miles apart, acting together to form a single radio dish. Their goal is to detect and triangulate the location of radio bursts emitted from the sun’s outer atmosphere.
PHOTO: NASA JPL/SDL
ILLUSTRATION: Scott Hyde
Studying the sun from Earth
While missions like Parker and Solar Orbiter study the sun from space, data captured during the eclipse offers the opportunity for a different perspective that could uncover new insights about the sun’s inner workings and how it shapes the rest of the solar system, including the Earth.
One of the efforts that gathered that data during the April 8 eclipse is called Coronacast. Composed of five U-M graduate students in climate and space sciences and engineering and two graduate students from the University of Texas at Arlington, the Coronacast team aims to complement Parker’s work and help build a better understanding of how plasma and magnetic fields behave inside the sun.
Welling, who is one of Coronacast’s organizers, explains that the team uses computer models to predict the corona’s shape and size based on theories of how the corona is heated and how its magnetic fields fluctuate. The eclipse offered the team a rare opportunity to compare their model to real-world observations.
“Right now, we don’t know how to turn the dials on most of the parameters in our models that describe how plasma in the corona behaves, and the eclipse could help us learn where to set those dials,” he said. “The more we understand the real system, the more we can include real-world processes in our space weather forecasting tools.”
On April 8, as crowds in Indiana were staring into the sky, the Coronacast team was holed up in a classroom at the University of Texas Arlington, along the eclipse’s path. They teamed up with three photographers from the International Astronomical Union who planned to take photos during the eclipse. The team hoped to compare the real-world photos to projections of the eclipse created by four different iterations of their computer model. While all four models generated plausible predictions of the corona’s behavior during the eclipse, only real-world data could show them which prediction most closely matched reality.
Sadly, the weather leading up to the eclipse didn’t cooperate; with overcast skies predicted, the Astronomical Union team packed up their gear and headed out in search of clearer skies.
But Coronacast had another source of data to turn to: The Center for Astrophysics Harvard & Smithsonian and crew members from the National Center for Atmospheric Research. They had recently taken off in the National Science Foundation’s Gulfstream V airplane for a look at the corona from the sky.
The two teams had partnered up in the months leading up to the eclipse to share data; Coronacast’s models helped the Center for Astrophysics refine their eclipse mission plans to capture the best possible data, while the Coronacast planned to use that data to better understand the physics that govern the flow of heat and energy throughout the corona.
The team’s aerial vantage point held two big advantages over monitoring the corona from the ground. First, the plane’s speed enabled the team to keep up with the eclipse’s movement across Texas, extending their data-gathering time by nearly 50%. In addition, the plane’s altitude gave the team better access to infrared wavelengths that would be absorbed by the Earth’s atmosphere if measured from the ground.
As the plane flew into the path of the eclipse between Dallas and Austin, the team used their Airborne Coronal Emission Surveyor (ACES) device to measure the corona’s infrared light. Each type of particle in the corona’s plasma absorbs and emits characteristic frequencies of infrared light, and the amount of light emitted at each frequency depends on the plasma’s temperature and density as well as its magnetic field activity.
Since the eclipse, the Coronacast team has used that data to generate a picture of the corona’s behavior during the eclipse and compare it to output from their models. They’ve studied the positions of holes in the corona, which appear as darker regions in images of the sun’s atmosphere, as well as cones of plasma called “helmet streamers” for their resemblance to 19th-century military helmets.
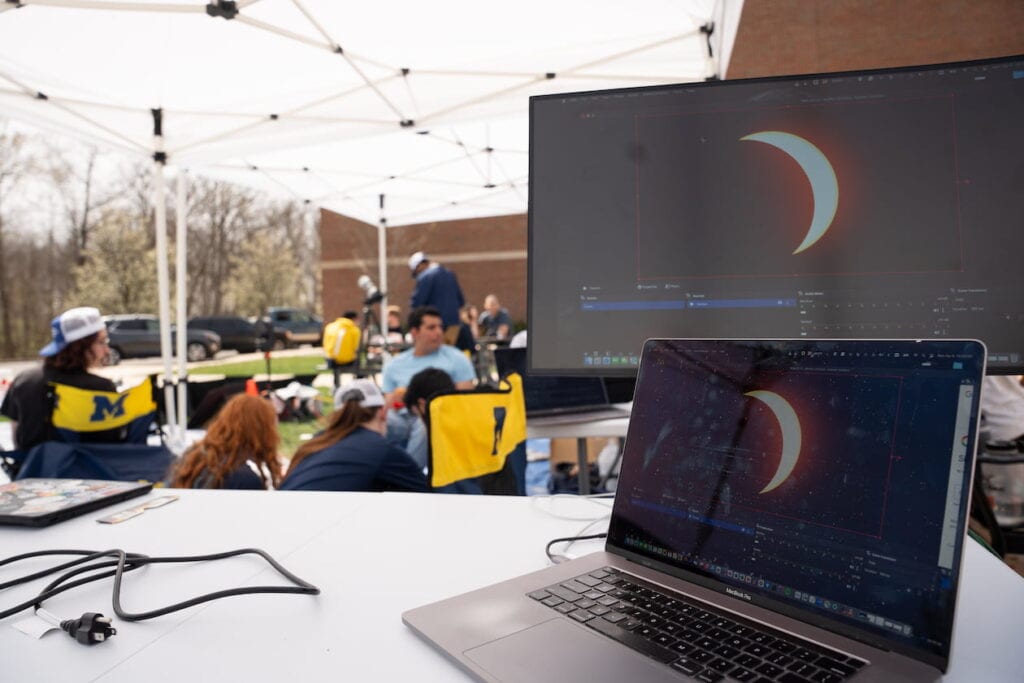
Model meets reality
The eclipse gave U-M’s Coronacast team a rare opportunity to compare their predictive model to real-world observations.
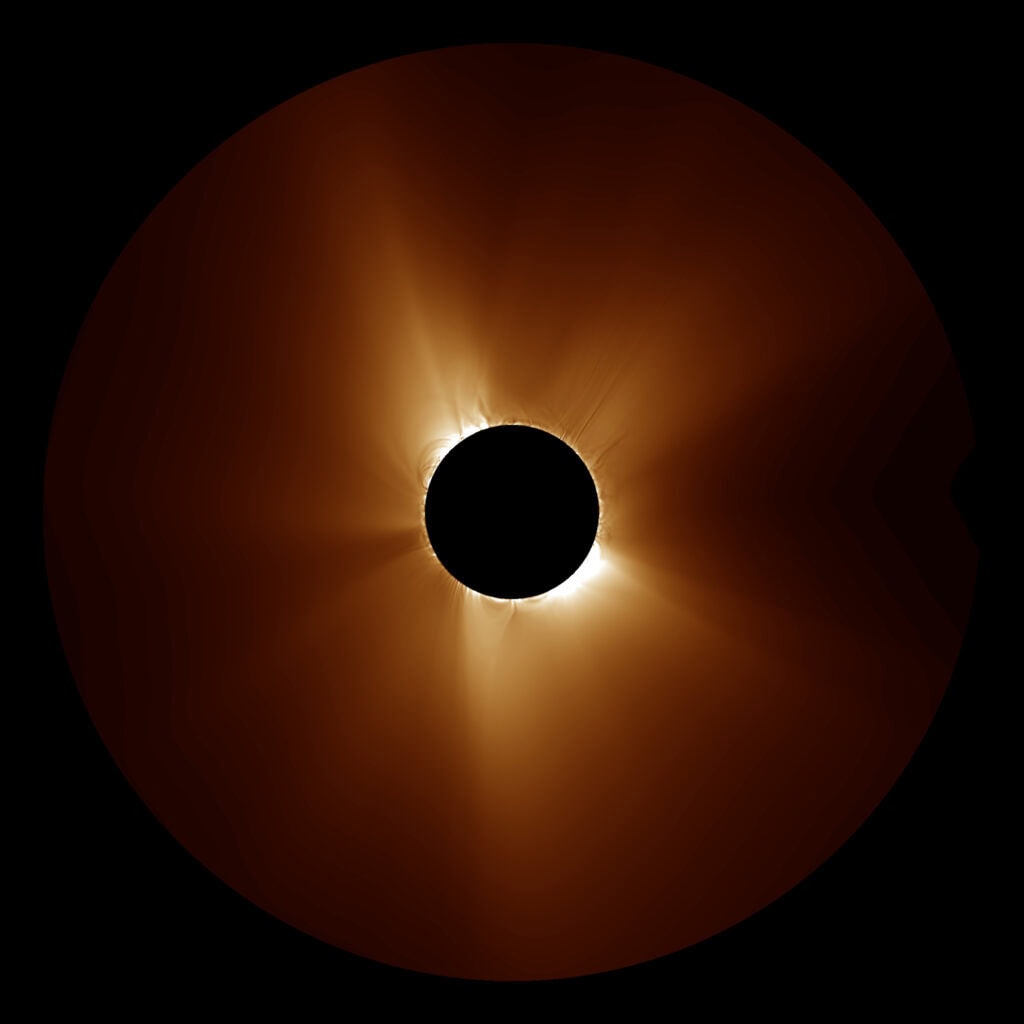
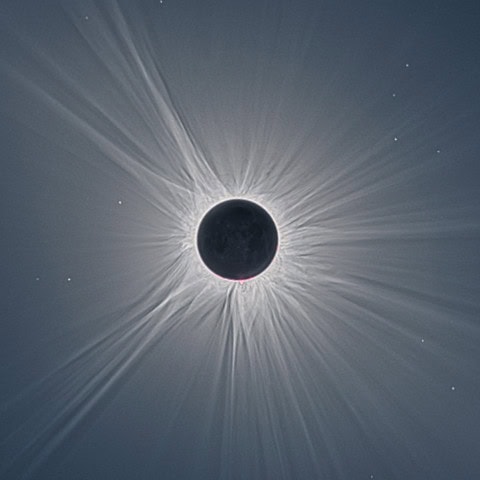
While it’s still early in the process, qualitative observations are already showing clear winners among the four models, with one proving to be particularly adept at predicting the locations of holes and helmet streamers. The team is currently performing quantitative analysis of the data, which they plan to incorporate into an academic paper as of press time.
“Down the road, when data on the corona’s temperature starts to come in, we’ll have an even better sense for how accurately we were able to simulate the temperature of individual features,” Welling said. “Solving the coronal heating problem would mean that we’re going to be able to start making meaningful forecasts of space weather from the sun to the earth more consistently, but getting to this point already has been great.”
“
Right now, we don’t know how to turn the dials on most of the parameters in our models that describe how plasma in the corona behaves, and the eclipse could help us learn where to set those dials. The more we understand the real system, the more we can include real-world processes in our space weather forecasting tools.
Listening for answers
Back at Taylor University, the SunRISE Ground Radio Lab students continued to watch for coronal mass ejections. They would appear as spikes in the lines of radio data that snaked across their screens, fed by cables that led to a series of radio antennas staked into a nearby lawn. Also tuning in were the 17 SunRISE Ground Radio Lab high schools across the country, the massive antenna at NASA’s Deep Space Communications Complex in southern California and a network of HAM radio operators who had volunteered to help.
“The moon’s shadow will impact all the wavelengths, from X-rays to radio waves, and each wavelength shows a different part of the sun,” said Shirsh Lata Soni, a postdoctoral research fellow in climate and space sciences and engineering who is coordinating work with the SunRISE high schools. “By combining it all together, we get the entire picture of the sun from the corona down to the surface. And with the moon slowly covering the sun, we can get our observations pixel by pixel in very fine resolution.”
The SunRISE team didn’t capture any coronal mass ejections during the eclipse, but they did capture a trove of data that will help researchers better understand the sun’s radio activity and how it might help them predict future space weather events.
“You never see an intense radiation storm near Earth without the sun first giving off low-frequency radio bursts, sometimes 10 minutes or an hour before it gives off the high-energy radiation,” said Kasper, who is the principal investigator of SunRISE. “Sometimes we get a radio burst without getting radiation, and we think that might be because it’s coming from the other side of the coronal mass ejection and Mars is getting zapped with radiation. We want to image those low-frequency radio waves to be able to see where in the coronal mass ejection it is coming from.”
In addition to the scientific data gleaned by the SunRISE Ground Radio Lab, the project is giving high school students around the country their first opportunity to participate in scientific research and master the assembly and operation of scientific equipment. Akhavan-Tafti believes that experiences like these are just as important as the radio data they’ve gathered.
“Our goal is to expose students of all backgrounds to space sciences and engineering early in their careers, with the hope of inspiring tomorrow’s space scientists, engineers, entrepreneurs and decision-makers,” Akhavan-Tafti said.
If the experience of the students at Hamtramck High School is any indication, Akhavan-Tafti’s plan appears to be working. The students formed a new astronomy club specifically to contribute to the SunRISE Ground Radio Lab. Madeha Rahman, the head of the club, organized the efforts to get her school’s antenna running and even convinced her parents to set up a second antenna at their house.
“I really love doing it,” said Rahman. “The most amazing part of it was when I got to know that the antenna was working. When they told me, ‘You are good, it is very good data,’ I was really happy.”
Fellow students Aymen Saleh and Mohammed Miah also joined the club once they saw the chance to get hands-on. Since then, their interest in physics and chemistry has skyrocketed.
“It’s the first time that I’ve seen them enjoy a long-term project,” said Natalia Olar, a chemistry teacher at Hamtramck High School. “My students are the greatest thing in the world to me, so seeing them get involved and learning and being curious about the world beyond what we see has been the most rewarding part of the project.”

Students from Ann Arbor’s Skyline High School also participated, including juniors Liana Zhou and Lillian Cui. The antenna Zhou set up at her house captured the project’s first radio burst from a coronal mass ejection on April 23, 2024.
“I think that as we’ve continued to work on GRL, I’ve only gotten more interested in solar radio bursts and space science because we’ve learned more about how it connects to us in our everyday lives,” Cui said.
Zhou and Cui both continued their work with SunRISE as interns with Soni through the summer of 2024. Rahman has continued to provide data from her own personal antenna, and she is eager to start a new radio astronomy club when she starts college at Purdue University.
“It just feels really rewarding and promising that our contributions and our engagements are actually going to make a difference in this project, and hopefully make a difference in this field,” Zhou said.
“
It just feels really rewarding and promising that our contributions and our engagements are actually going to make a difference in this project, and hopefully make a difference in this field.