New energy
Once derided as “forever 30 years away,” fusion energy has a new swagger. Will it last?
Written by Madeline Diehl
When Annie Kritcher jolted awake in the early hours of Monday, December 5, 2022, she went straight for the cell phone next to her bed. As soon as she entered the access codes for her secure government account, she saw the message she’d been hoping to see for months:
“I think we just got gain.”
Contents:
Kritcher (BS NERS ’05), the lead experimental designer at the Lawrence Livermore National Laboratory’s National Ignition Facility (NIF), couldn’t believe her eyes. A little after 1 a.m., the experiment she designed had just achieved fusion ignition, something scientists had been trying—and failing—to accomplish for some 60 years. For the first time, a self-sustaining fusion reaction in a peppercorn-sized fuel pellet gave off more energy (3.15 megajoules) than the amount of laser energy that had been injected into it (2.05 megajoules). She had changed the field of nuclear fusion literally overnight.
The achievement is a monumental step forward in fusion energy research, which for decades has been chasing the dream of harnessing the same phenomenon that powers the stars to provide humans with abundant, clean, carbon-free energy.
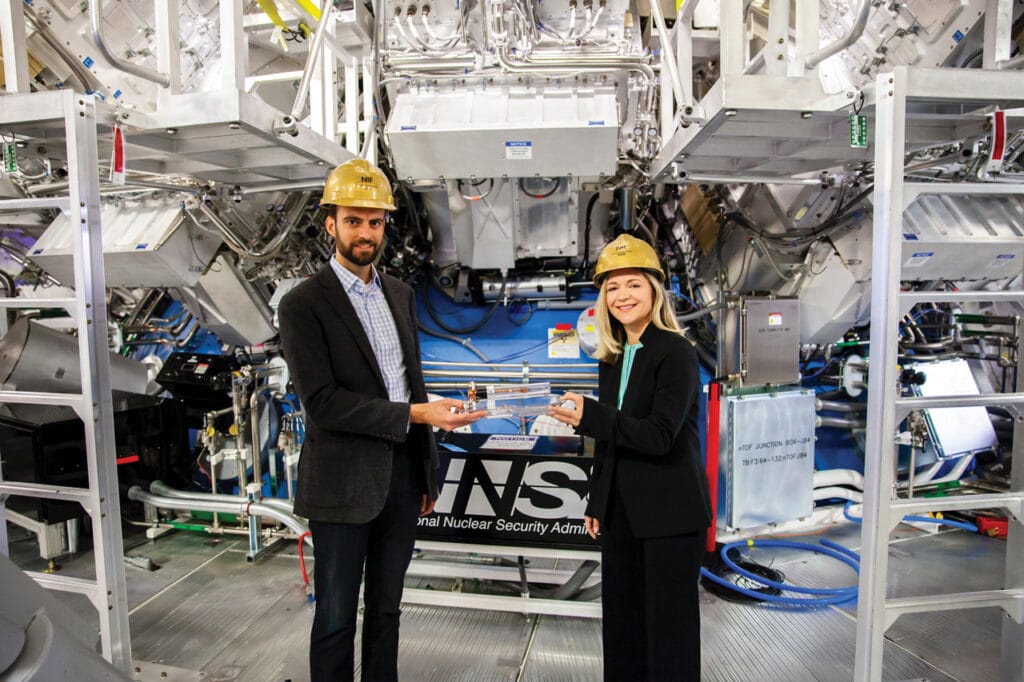
Fusion is, in essence, the opposite of the fission that powers today’s nuclear power plants. Instead of splitting heavy atoms, it fuses two light atoms into a single atom that’s lighter than the sum of its predecessors—turning the extra mass into huge amounts of energy. There’s no long-lived radioactive waste, and unlike fission, there’s no risk of a meltdown.
The field has seen a flurry of activity in the past several years, driven by the urgency of addressing climate change and by the potential investor payoff of a lucrative new energy source. Advances are coming both at government facilities like NIF and at dozens of private startups.
“Fusion is, in essence, the opposite of the fission that powers today’s nuclear power plants.“
Kritcher’s particular advance—designing an experiment that achieved the first net energy gain from a self-sustaining fusion reaction—was hailed by U.S. Department of Energy Secretary Jennifer Granholm as “one of the most impressive scientific feats of the 21st century.” It also landed Kritcher a spot on TIME magazine’s list of the 100 most influential people of 2023. Kritcher is also featured in the Fall 2023 Michigan Engineer issue’s “Alumni Notes” section.
Too excited to sleep after learning of her achievement, Kritcher headed into the lab early the next morning and found a party atmosphere. Some people were hugging, jumping up and down and screaming, but Kritcher kept her cool.
“I’m not a big jump-up-and-down person,” she said. “I feel my emotions more internally. But I was laughing and tears were welling up in my eyes.”
But the party didn’t last long. “There was a lot more work ahead of us, and we had to work night and day to vet the diagnostics and make sure our analysis was correct,” explained Kritcher. “We had to make sure we didn’t have a ‘gotcha.’”
“Gotchas”—experimental results that can’t be reproduced, prematurely reported findings and apparent breakthroughs that wither under scrutiny—have plagued fusion energy since the 1950s. They’ve led many scientists to dismiss the field as a technology that’s “forever 30 years away” and not worth the time and money to pursue. Perhaps as a result, many of the scientists who have been pursuing fusion for decades share Kritcher’s cautious demeanor.
Fusion research on North Campus
Among those scientists is Ryan McBride, a U-M professor of nuclear engineering and radiological sciences and director of U-M’s Plasma, Pulsed Power, and Microwave Laboratory (PPML), which focuses on inertial confinement fusion. “Inertial confinement” refers to the method used to corral plasma, an extreme state of matter made of positive ions and free electrons with properties distinct from solids, liquids or gases. Containing and controlling the plasma that results from the massive heat and pressure inside a reactor is one of fusion’s key challenges.
While the NIF solves the problem by squeezing plasma with hundreds of laser beams, PPML uses a phenomenon called a Z-pinch, which applies a strong electrical current to plasma, generating a magnetic field that squeezes it into a narrow line down its z-axis.
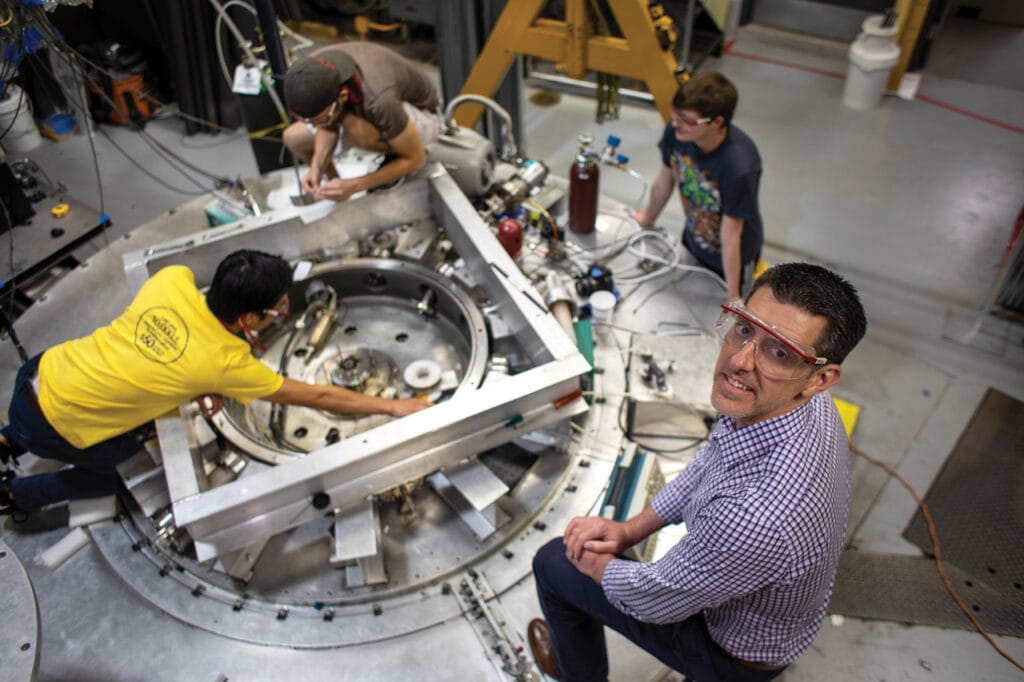
PPML uses a custom-built device called the Michigan Accelerator for Inductive Z-Pinch Experiments (MAIZE) to drive as many as 30 fusion experiments per day. But like the device in the NIF, it isn’t focused on generating electricity. Instead, its research is geared toward understanding the fundamental physics of plasmas. Understanding those physics is important for maintaining U.S. nuclear weapons stockpiles, and it could also help researchers who are working to develop fusion power.
Like many of his generation, McBride entered the fusion space in the early 2000s, at a time when the field was getting far less attention. Climate change was not yet in the global consciousness as it is today, and fusion’s tendency to make fools of its proponents left many scientists reluctant to get behind it. Researchers who did recognize the field’s importance had fewer places to do their work, mostly government labs that were researching fusion for reasons other than generating electricity. McBride started his career in the weapons stewardship program at Sandia National Laboratories in 2008 before joining U-M in 2016.
“I initially got into this field because of fusion energy,” he said. “The national labs had (and still have) some of the best fusion research machines available. But the research programs on these facilities were (and still are) mainly for nuclear weapons stockpile stewardship.
I figured, well, while the U.S. is doing the stockpile stewardship research, we’re also getting more experience to potentially build a power plant down the road.”
In July 2023, McBride took charge of the Center for Magnetic Acceleration, Compression and Heating (MACH), which moved to U-M from Cornell University. MACH will study the physics of magnetically compressed and heated plasmas, as well as the physics to aid in the construction of larger and more efficient fusion machines. The multi-university center is funded by a five-year, $14.5 million grant from the National Nuclear Security Administration.
While McBride celebrated Kritcher’s achievement, he believes that it will be decades before fusion supplies significant amounts of energy to the grid. He points out that the NIF is only equipped to ignite a target capsule about once a week, and grid-scale electricity would require many capsules exploding many times per second.
“I believe we’re in a period similar to when fission scientists were still doing bench-top experiments in their labs,” he said. “It’s just such a big leap to go from demonstrating ignition to getting fusion power on the grid. Are we 20 years away? I don’t know, it could be another 50 years easily.”
McBride and many of his contemporaries see progress toward fusion as a steady, linear process that begins with fundamental physics, then progresses through reliably controlling fusion, then building larger reactors, then connecting them to electrical generators, and finally, one day, integrating it all into the grid.
The world’s biggest science project
That process has been plodding ahead for the past six years in France as ITER, a 35-nation collaboration, has been building a fusion reactor known as a tokamak. Originally developed in the Soviet Union in the 1960s, the tokamak concept has for decades been considered the most likely path to the extraction of energy from fusion.
While ITER’s tokamak is by far the world’s largest, there are more than 50 others around the world working to crack the code of how to generate electricity from fusion.
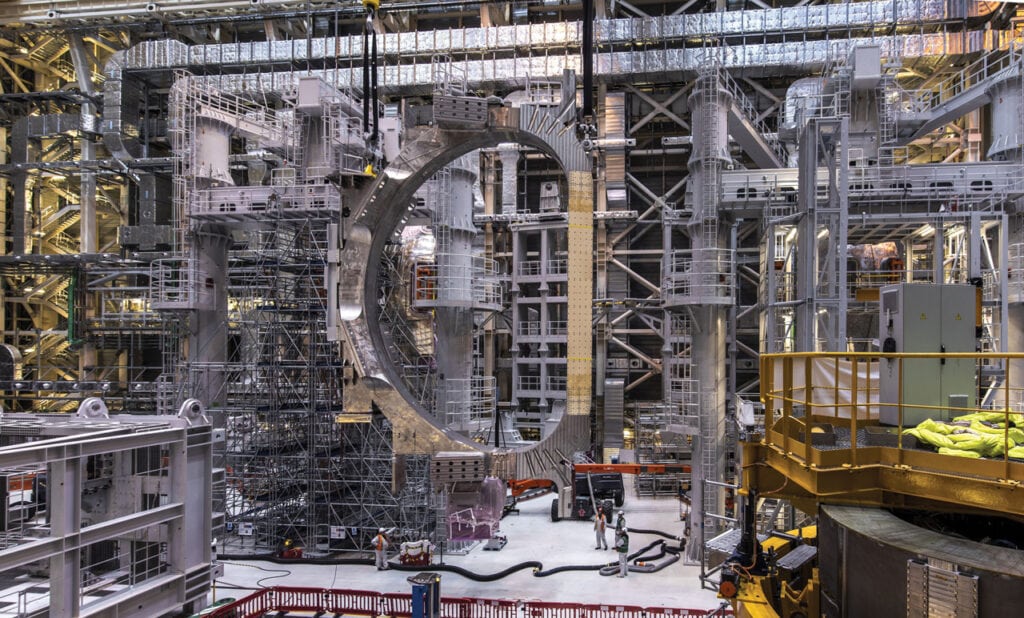
A tokamak uses powerful magnets to confine a fusion reaction inside a doughnut-shaped chamber. Like a campfire that must be hot enough to ignite fresh logs, the plasma has to be hot enough to perpetuate a self-sustaining fusion reaction. This means that a tokamak has to withstand the magnetic and physical acrobatics of a burning plasma at colossal temperatures of around 270 million degrees Fahrenheit—
hotter than the interior of the sun.
Construction on the ITER tokamak began in Saint-Paul-lès-Durance, France in 2017 with a budget of $6.3 billion. It’s scheduled to be completed in 2025, though documents recently revealed by Scientific American suggest that that timeline may not be realistic. The budget has ballooned as well, to over $22 billion.
The goal is for the 23,000-ton machine to return a ten-fold energy gain and generate 500 MW of output power, though the power will be generated solely as heat and won’t be turned into electricity. The project has been called “the most expensive science experiment of all time” and the most complicated engineering project in human history.
Considering the massive scale and budget of ITER’s tokamak, it’s easy to see why McBride, along with most other fusion experts, believes that we’re unlikely to see significant amounts of fusion energy on the grid until at least 2050.
Types of Fusion Machines
Illustrations by Stephen Alvey
TOKAMAK
A tokamak, such as the one being developed by
ITER, heats deuterium and tritium fuel to form a plasma, then uses powerful magnets to confine that plasma inside a doughnut-shaped vacuum chamber. ITER’s tokamak is designed to contain a plasma more than 40 feet in diameter.
Toroidial field coils run the long way around the chamber, squeezing the plasma horizontally, while the central solenoid creates a second magnetic field running the short way around, squeezing it vertically. The outer poloidial coils shape the plasma further, helping to create the conditions for a self-sustaining fusion reaction.
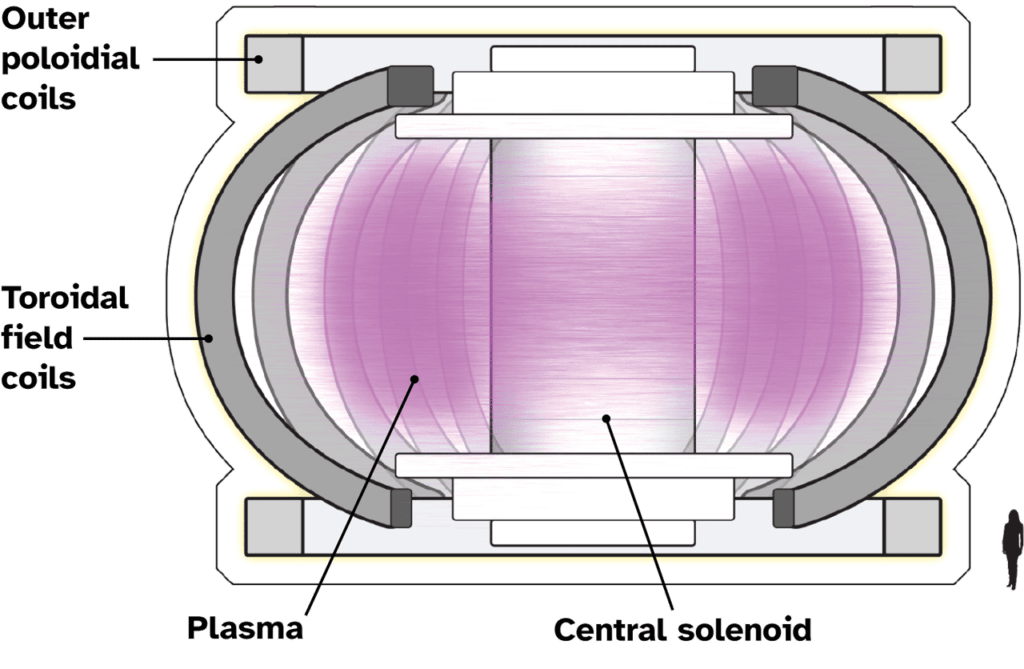
Z-PINCH
Instead of using external magnets to confine a plasma,reactors like the PPML’s MAIZE machine use the Z-pinch principle. An ultra-powerful electrical field is applied to the plasma, causing it to generate a magnetic field that squeezes it into a fine line.
The MAIZE machine uses 80 high-voltage capacitors and 40 switches arranged in a 9.8-foot diameter circle around a vacuum chamber. The capacitors discharge their energy through a small column of deuterium gas in the center of the vacuum chamber,
compressing it on the z-axis (a “Z-pinch”) and progressing toward plasma densities and temperatures that could one day induce a
fusion reaction.
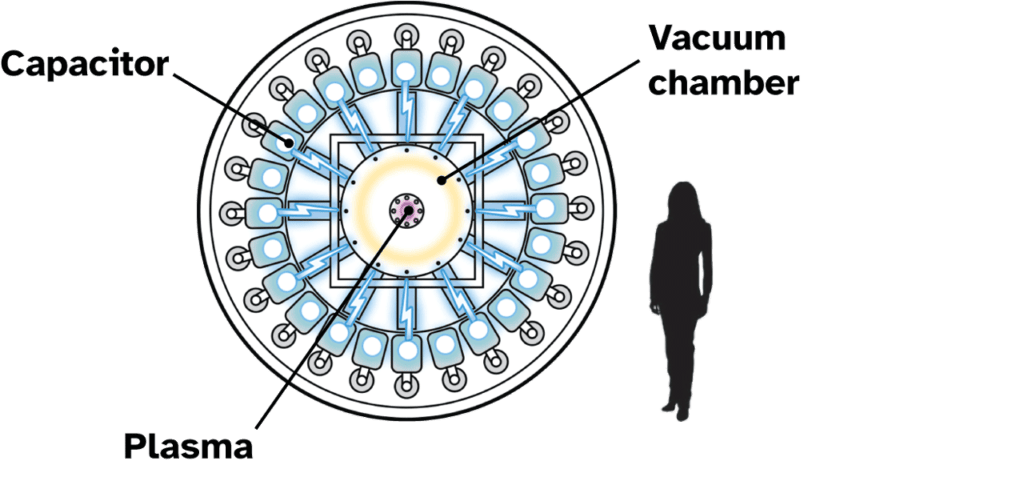
Plasma accelerator
Several fusion startups, including Helion Energy, use plasma accelerators that form two plasmas at opposite ends of a chamber, then use magnets to contain and accelerate them toward a smaller space at the center. This generates the heat and pressure required for a pulsed fusion reaction. Helion’s reaction chamber measures 40 feet from end to end.
This example shows Helion’s proposed process, which would use metal coils to harness fluctuating magnetic fields from the expanding plasma reaction, directly generating electricity. Other plasma accelerators would harvest heat from the reaction, producing steam to turn a generator’s turbine.
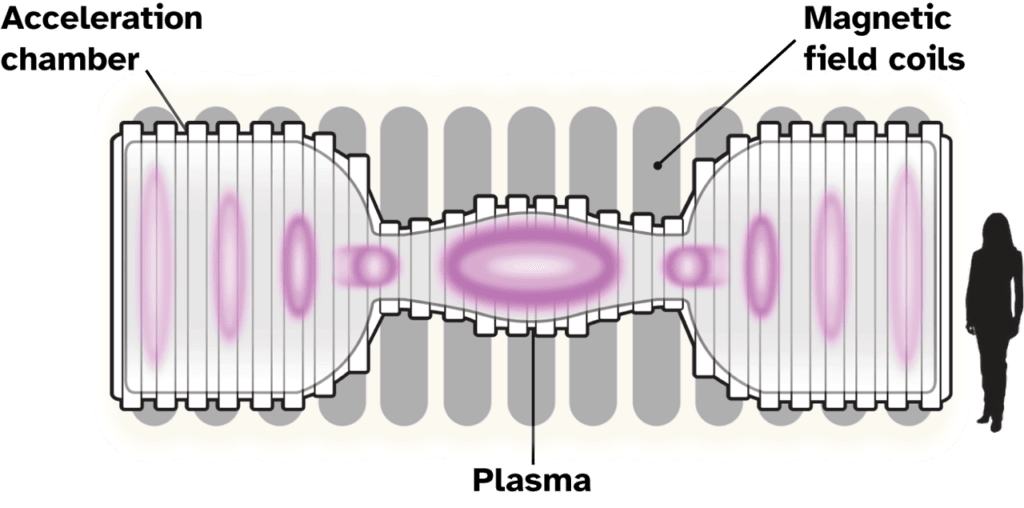
The disruptors
But a collection of private fusion startups—more than 30 of them as of an October 2021 survey by the Washington D.C.-based Fusion Industry Association—has other ideas. They’ve attracted more than $5 billion in funding from the likes of Google, Bill Gates, Jeff Bezos, Shell Oil Company and others.
They’ve raised that big money by making big promises—some even pledging to have fusion power on the grid before the end of the decade. They’re also bringing a glossy, go-fast vibe to the fusion space that could be straight out of Silicon Valley. One of the most well-funded fusion startups, Helion Energy, was founded by Michigan Engineering alum David Kirtley (BSE Aero ’01, MS AeroE ’05, MSE NERS ’05, PhD Aero ’08).
“Yes, I am wearing pink shoelaces,” says Kirtley from the other end of a Zoom line, raising his dark, bushy eyebrows. “And all of our furniture is pink. Here, check out this chair.” He swivels his webcam to reveal an office chair clad in a juicy shade of fuchsia.
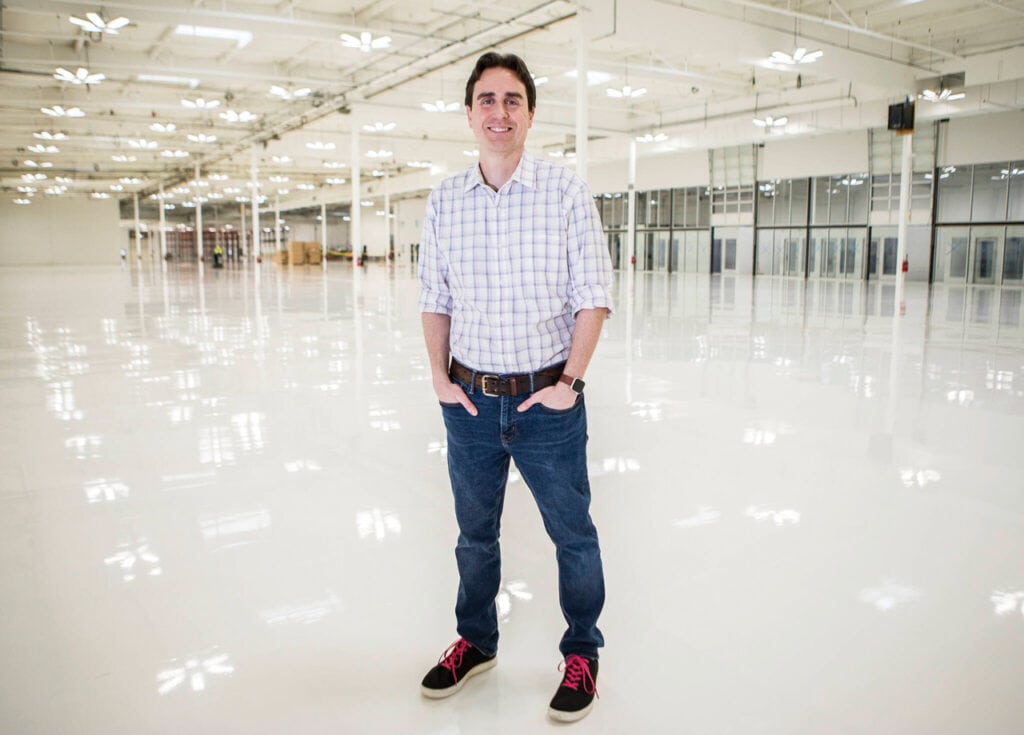
Kirtley explains that Helion’s décor was inspired by the pinky glow that emanates from its particular type of fusion reaction.
Helion is betting that it can generate fusion power with a series of pulsed fusion reactions rather than a self-sustaining reaction—more like a string of firecrackers than a campfire. This string of reactions would take place at lower temperatures than those inside a tokamak, sidestepping some physics challenges and, the company claims, enabling smaller, cheaper fusion generators that could fit onto a train car or a tractor trailer.
Kirtley claims that Helion will demonstrate electricity production by 2024, and the company has a contract to begin selling power to Microsoft in 2028. The company’s system uses two separate plasmas at opposite ends of a 40-foot chamber. The plasmas are accelerated by magnets to one million miles per hour, colliding in the middle of the chamber in an energy-releasing fusion reaction.
Once that reaction happens, another key difference in Helion’s system comes into play. Other fusion efforts to date have focused on extracting heat from the reaction and using it to produce steam and turn a generator’s turbine—much the same way electricity is generated by fossil fuels today. Helion, however, claims that its system can instead use magnetic field fluctuations caused by the expanding plasma to directly generate electricity through Faraday’s law.
Because the direct-generation system would eliminate the need for a separate power plant, Helion is confident that it could generate power at a significantly lower cost than its competitors.
“We started out like everybody else, but we realized that it didn’t make sense,” explained Kirtley. “Using deuterium and tritium produces a lot of heat, but then you have to convert that heat into electricity,” he continued. “We got to a place where we were asking: are we even using the right fuel? Why don’t we skip the heat cycle and just make electricity directly?”
“Yes, I am wearing pink shoelaces.”
Helion also uses a different fuel than the deuterium and tritium used by ITER and all other government labs. The company has patented a process that purports to use deuterium and helium-3 to achieve fusion reactions. Helium-3 is an ultra-rare form of helium with two protons and one neutron, and until recently, it was found only on the moon. But Helion has patented a process that enables it to manufacture helium-3.
Manufacturing an ultra-rare form of helium may sound far-fetched,
but then again, the traditional deuterium and tritium combo has its
own set of problems. Deuterium can be obtained from seawater in
essentially unlimited quantities. Tritium, however, is tricky—the global
stockpile of the ultra-rare element amounts to about 25 kilograms.
Tritium could theoretically be manufactured in fusion reactions, but the
idea has never been tested in a reactor.
If that all sounds a bit vague, that’s because, well, it is. Much of the technology being developed by Helion and other private fusion companies is proprietary, so it can be difficult to determine exactly how the projections square with reality. In addition, the private companies are pursuing an “everything, all at once” approach that differs sharply from the steady, linear progression of more traditional fusion research.
Helion claims that it has built six prototypes, each focusing on a particular part of its technology, and it’s often working on several at once. Its latest, Trenta, was completed in 2020. The company claims that Trenta has completed almost 10,000 high-power fusion pulses and operated under a vacuum for sixteen months. Trenta was also the first privately built fusion machine to reach plasma temperatures of 180 million degrees Fahrenheit, hot enough to generate commercially viable levels of electricity. The company expects to complete its latest prototype, Polaris, in 2024.
“We do this because we think that it’s the best way to get our technology out to the world as fast as we can,” Kirtley said. “That means we need to be designing and building the latest prototype while we’re also designing the one that comes after that. At the same time, we’re working in parallel on the supply chain because that sets our timeline as much as anything else, including engineering. So we constantly have to be focusing on building our business, too.”
“If you have good ideas and things don’t work out, that’s just science.”
Other private fusion startups use wildly different approaches; California-based TAE Technologies, for example, is focused on achieving fusion with hydrogen-boron fuel, while Boston-based Commonwealth Fusion Systems is working on simpler, smaller versions of tokamak technology. Zap Energy, based in Seattle, is pursuing a technology that would use a Z-pinch to contain plasma, the same basic principle used in U-M’s PPML lab. The through line is smaller, cheaper, lower-temperature technologies that don’t require the massive scale of ITER’s tokamak.
Power tomorrow, jobs today
While private fusion companies have yet to produce any electricity, they’re already generating opportunity for a new generation of fusion scientists. Today’s grads have their choice of good-paying, energy-focused jobs in the field, funded by fat checks from investors.
Among them is Brendan Sporer (PhD NERS ’23), who completed his PhD in McBride’s lab. He interviewed at Helion, TAE Technologies and p Energy. Ultimately, he chose to get behind TAE’s approach, as well as its sunny southern California location.
Sporer is confident that the world is on the precipice of a fusion-driven future, and he believes that new computational tools and new materials make today’s fusion research fundamentally different from that of past decades.
“We now have technological advancements that have changed the game,” said Sporer. “I think new computational tools are the biggest one, where we now have the ability to simulate an entire fusion reactor before we build it. This wasn’t possible 20 years ago.”
He hesitates for a moment. “Or maybe I am being like everybody else has always been?” he asks rhetorically. “This may be what everyone has said in their time.” Then his voice becomes confident again. “But no. I’m sure that today is different from five or ten years ago. We all have to go in with healthy skepticism. This is going to be a lot harder than people are making it sound. This is going to be extremely hard. But I think we’re in the homestretch, and I’d like to be there when it happens.”
The government is also buying into private fusion; on March 17, 2022, Granholm announced $50 million in new grants to fund two public/private partnerships. The partnerships will work to build a demonstration fusion power plant in the 2030s. May 2023 saw the announcement of $45 million in additional funding for eight private fusion companies including Commonwealth Fusion Systems and Zap Energy. The goal is in line with President Biden’s energy policy, which calls for the nation’s power generating sector to be carbon-neutral by 2035.
Fail fast, fail early
Other researchers, however, are concerned that the new optimism could fuel fusion’s long reputation for over-promising. That includes Carolyn Kuranz (PhD Applied Physics ’09) a U-M associate professor of nuclear engineering and radiological sciences. Still, she’s pleased to see fusion receiving an influx of interest and money. It’s a welcome contrast with the state of the industry during the era when she and McBride finished graduate school.
As a graduate student, Kuranz worked at the Omega Laser Facility at the Laboratory for Laser Energetics in Rochester, New York, doing work related to nuclear stockpile stewardship before joining U-M in 2009 to work in its Center for Radiative Shock Hydrodynamics (CRASH). There, she studies the physics of hot, dense plasmas similar to those inside fusion reactions, as well as those that exist naturally in space. Like McBride, her work is not directly tied to using fusion to generate electricity, but she’s uncovering new knowledge about the fundamental physics that could move the world closer to fusion power.
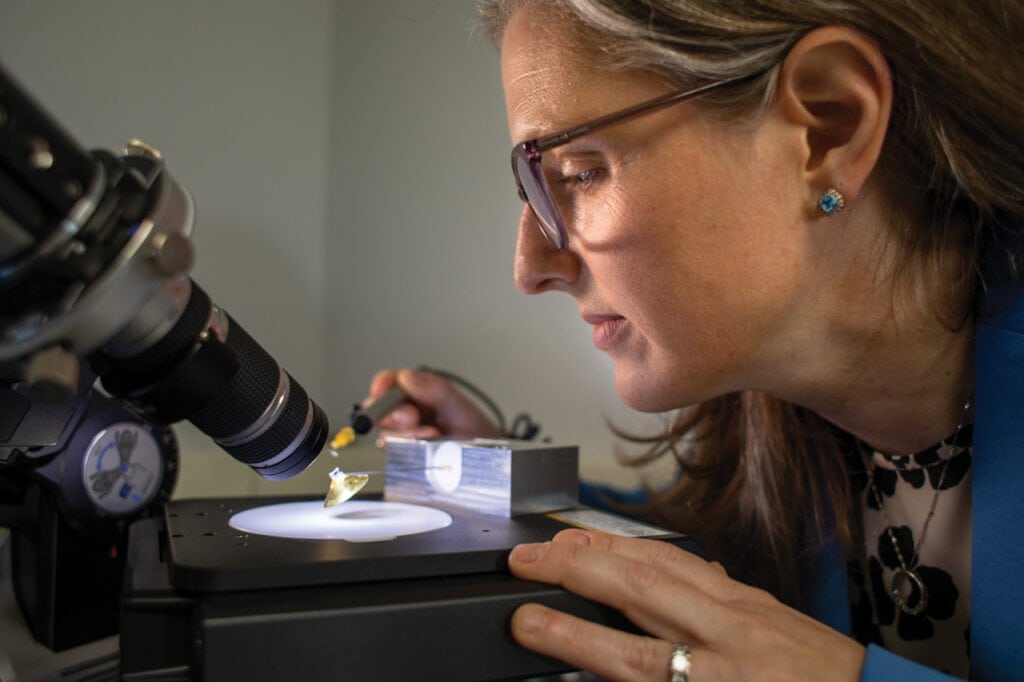
These days, many of her students are interested in working for private fusion startups. Though she tells them to exercise caution, she also encourages them to enjoy the fact that they’ve come up in a boom time for fusion.
“As scientists we always need to be curious and hopeful,” she said. “We do need to balance that with realism and pragmatism, but if we’re not willing to take risks, we wouldn’t be anywhere. We wouldn’t have computers, flight, smart phones, any of these things.”
“The fact is, many of these companies will fail. But I don’t think there is anything wrong with a company if they do good science and fail. If you have good ideas and things don’t work out, that’s just science. This is the time for us to take a chance on fusion, because there’s no way society can rely on renewables alone … and we’re running out of time.”
Written by Madeline Diehl