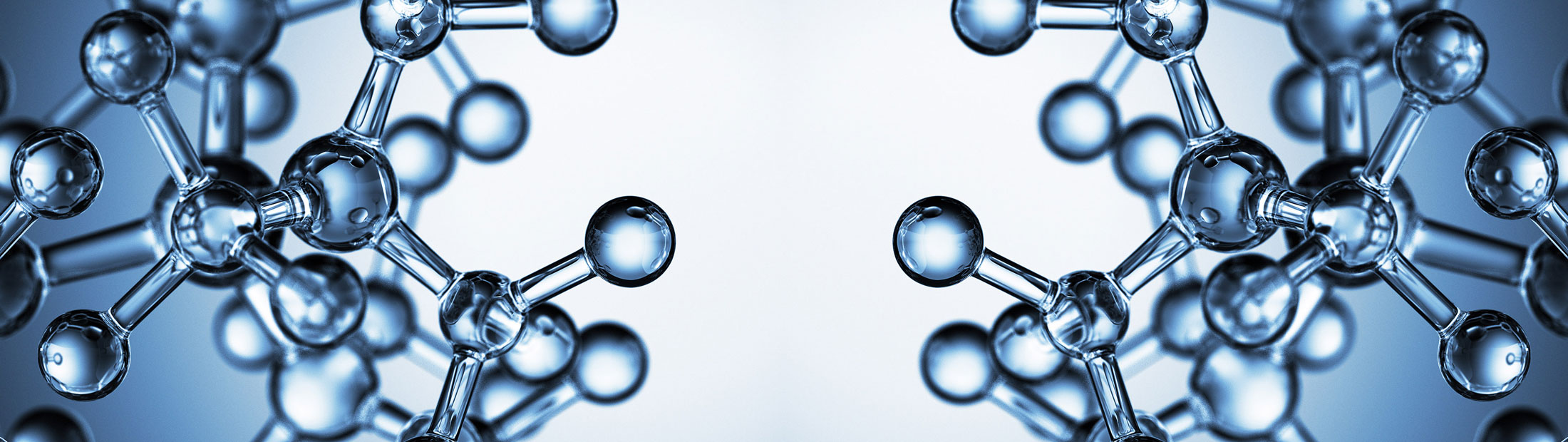
Nanoparticle-based, bio-inspired catalyst could help make more efficient reactions affordable
Chemical processes usually give us both mirror image versions of a molecule when we want only one.
Chemical processes usually give us both mirror image versions of a molecule when we want only one.
Many chemical compounds come in two versions that are mirror images of one another, as are our right and left hands. This property is known informally as “handedness” and more formally as chirality. Biological systems, including our own bodies, often show a preference for interacting with the right-handed version of a molecule over the left or vice versa, but when chemists make chemical compounds, the results tend to be evenly split between the two versions of the molecule.
A chiral catalyst can change that, driving the reaction toward one handedness over the other. Existing catalysts with this capability are hard to make and use, so they are impractical for many applications. But a new catalyst built from nanoparticles could change that.
This catalyst, which mimics the way that biological cells perform chiral chemistry, is at least 10 times better at selecting a particular version of a chiral molecule than earlier catalysts of this type. Nicholas Kotov, the Joseph B. and Florence V. Cejka Professor of Engineering, led the team that designed and tested it. He answered some questions for the Michigan Engineer.
Most medicines are chiral. Even though they are identical atomically and nearly identical structurally, the two versions of the molecule (known as enantiomers) have markedly different biological activities. Often, one is effective while the other does nothing—this is why 90 percent of chiral medicines contain both enantiomers.
But in some cases, the wrong handedness is toxic. We come by this knowledge in part through a tragedy: In the late 1950s, the anti-nausea drug Thalidomide caused thousands of stillbirths and limb deformities because one of the enantiomers was harmful to developing fetuses.
While enantiomers can be separated, the process is costly and tremendously increases the price of drugs that need separation. A chiral catalyst can bias the synthesis of a drug toward the therapeutic chirality, reducing the price of the medicine. Potentially, chiral catalysts could also reduce the cost of drugs that we don’t currently separate—essentially halving the amount of active ingredient needed to provide the same effect.
Besides drugs, chiral compounds are needed for liquid crystals used in displays. Chiral catalysis makes it possible to produce essential chemicals needed for these technologies faster and cheaper.
Chiral catalysts today have been optimized for performance rather than usability. Many of them can produce left- or right-enantiomers almost exclusively, but they are pretty hard to make. They are fairly sophisticated chiral compounds made using multi-step organic synthesis under stringent conditions, and these compounds need to be carefully purified.
The catalysis typically happens on chiral centers around single atoms, which are often expensive noble metals like platinum and palladium. And they’re often not reusable.
Then when you go to use them, they are sensitive to their environments, which limits the range of conditions that they can work under. These molecules can break down if the environment becomes hot or acidic, for instance. Furthermore, they typically require toxic organic solvents. We would much rather do these reactions in water, which is safer and much less costly.
Also, typical chiral catalysts fall apart under UV light, so they use heat to produce reactions. Because heat is less energetic, it takes more steps to go from starting materials to the final product. With the greater concentration of energy in light, we may be able to skip intermediate steps, which cuts down on both energy use and chemical waste.
Our catalysts have conceptually different design inspired by biological organelles known as membrane-less compartments, such as those that perform photosynthesis in some bacteria. They are spherical in shape and about 100 nanometers in diameter.
Earlier biomimetic chiral catalysts have terrible enantioselectivity—about one to two percent. But these date back to the 1970s, well before the advent of today’s nanotechnology, so we thought that we needed to give it a go.
We explored the preparation of chiral catalysts built from inorganic nanoparticles, made from semiconductors, metals and ceramics. These materials have high affinity to water, are highly stable, and are known to be catalytic. Such assemblies, known as supraparticles, are made of several hundred smaller chiral nanoparticles.
It turned out that we can assemble similar compartments in one step from inexpensive, inorganic nanoparticles made from zinc. Catalysis occurs in the gaps between the nanoparticles, where energy captured from the light frees up charge carriers (electrons and holes) that induce reactions. These gaps preferentially accept starting molecules of one chirality, biasing the reaction toward the desired chiral bond.
Our enantioselectivity is consistently above 20 percent, which means that we form the preferred bond 60 percent of the time rather than 50 percent of the time. That may not seem like much, but already it is technologically valuable because it reduces the cost of the intended product by 20 percent. And since they’re inexpensive, stable, and reusable, they could make sense economically even now for some processes.
We demonstrated the forging of a particular carbon-carbon bond that is essential for the synthesis of many medicines. This bond is present in the molecule dityrosine, so we made it by binding together two molecules of tyrosine, an amino acid made in the human body and also found in cheese. The same bond is found in molecules for liquid crystal displays.
Tyrosine amino acid appears in two chiral versions, the “L” version produced by mammals and the “D” version found elsewhere in nature. We found that our catalyst could preferentially select “L” or “D” tyrosine as building blocks, which determined the chirality of the dityrosine we produced. This suggests that we can design a catalyst to choose either bond geometry for other final products.
This study demonstrates that assemblies of chiral nanoparticles can carry out chiral catalysis differently than molecular catalysts. They represent a new toolbox that enables the synthesis of necessary enantiomers in energy efficient manner.
Beyond the efficiency of performing more direct chemical conversions, an exciting possibility is that we could harness the chirality of light itself to drive chiral reactions. Illumination with right- or left-handed photons can potentially improve the enantioselectivity further. It will be an important technological avenue to explore in the future.
In may be a long shot, but the same nanoparticle assemblies can potentially carry out the synthesis of peptide oligomers—short chains of amino acids much needed not only in medicine but also in agriculture and information technologies. These molecules could combat antibiotic-resistant bacteria, and they may be able to replace some pesticides if they can be made cheaply enough.